Translate this page into:
Biochemical and genetic analysis of Restless Legs syndrome (RLS): A pilot study
*Corresponding author: Dr. Rashmi Chowdhary, Department of Biochemistry, All India Institute of Medical Sciences, Bhopal, Madhya Pradesh, India. rashmi.biochemistry@aiimsbhopal.edu.in
-
Received: ,
Accepted: ,
How to cite this article: Verma A, Yadav AK, Goyal A, Gupta S, Jadhav AA, Kanwar JR, et al. Biochemical and genetic analysis of Restless Legs syndrome (RLS): A pilot study. Future Health. doi: 10.25259/FH_52_2024
Abstract
Objectives
Restless Leg Syndrome (RLS) is a neurological disorder characterized by an uncontrollable urge to move the legs, often worsening at night, leading to insomnia and discomfort. RLS is linked to genetic factors, defects in iron metabolism, dopaminergic dysfunction, and disturbances in the central opiate system. Specific genetic variants, including MEIS1, BTBD9, PTPRD, and MAP2K5/SCOR1, affect dopamine synthesis, iron transport, and neuroprotection. Research on RLS prevalence and its underlying causes in India is limited, highlighting the need for more in-depth genetic and biochemical studies to improve diagnosis and treatment.
Material and Methods
A hundred suspected RLS patients were screened at AIIMS Bhopal’s Pulmonary Medicine department. After obtaining consent, 36 confirmed RLS cases and age-matched healthy controls were enrolled. Blood samples were collected for fasting blood sugar (FBS), liver function tests (LFT), renal function tests (RFT), serum ferritin, and iron analysis using an auto-analyzer. Genotyping for single nucleotide polymorphisms (SNPs) was performed using ARMS-PCR.
Results
RLS significantly impacts daily life, contributing to insomnia, irritability, and other comorbidities. The study revealed lower iron levels in RLS patients, suggesting a potential secondary form of the condition linked to iron deficiency. Variations in serum iron, total iron-binding capacity, iron saturation, and ferritin further underscore the relationship between iron metabolism and RLS. Genetic analysis identified SNPs in genes such as MEIS1, TOX3, and PTPRD, potentially contributing to RLS. Although no specific at-risk alleles were prevalent, heterozygous mutations, particularly at the T allele, suggested a possible genetic predisposition that may exacerbate RLS.
Conclusion
This study supports the iron-dopamine hypothesis, linking RLS to brain iron deficiency that disrupts dopamine signaling. Genetic factors may further aggravate this disruption. Understanding the interplay between iron levels and dopaminergic function is crucial for effectively managing both primary and secondary RLS, aiding the development of better long-term treatment strategies.
Keywords
ARMS polymerase chain reactions
liver function test
renal function test
restless leg syndrome
single nucleotide polymorphism.
INTRODUCTION
Restless Leg Syndrome (RLS), also known as Willis-Ekbom disease, is a neurological disorder characterized by an overwhelming urge to move the legs, often accompanied by unpleasant sensations like pulling, burning, tingling, or itching. First identified by Ekbom in 1945, RLS remained underdiagnosed for years due to its diverse symptoms and absence of specific biomarkers. Symptoms typically worsen in the evening or night, interfering with sleep, and while legs are the most commonly affected, it can also involve the arms. Alongside motor symptoms, individuals often experience pain, cognitive disturbances, mood disorders, and sleep disturbances.1-4 RLS is classified into two types: primary and secondary. Primary RLS is believed to be hereditary with no known cause, while secondary RLS is linked to other medical conditions such as iron deficiency, kidney disease, and pregnancy.5
Diagnosis follows the criteria set by the International Restless Legs Syndrome Study Group (IRLSSG), which includes the urge to move the limbs, discomfort that improves with movement, worsening symptoms at night, and the absence of other underlying causes. The severity is assessed using the International Restless Legs Scale (IRLS), classifying patients as mild, moderate, or severe.6
RLS affects 1% to 5% of the population, with higher rates in older adults and women, and pregnancy being a significant risk factor. It is classified into two types: idiopathic (primary), without a clear cause, and secondary, linked to medical conditions like anemia, chronic liver disease, rheumatoid arthritis, multiple sclerosis, and obesity. Renal impairment and iron deficiency are strongly associated with secondary RLS, with iron-deficiency anemia increasing risk.7-16 Additionally, low brain iron causes hypoxia and demyelination, which further contribute to the disorder’s pathophysiology.3,4
Managing RLS requires assessing associated medical conditions and symptoms. Treatment options include pharmacotherapy tailored to the patient’s medical history, as severe RLS can severely impair quality of life and lead to insomnia.
The pathophysiology of RLS involves iron metabolism disturbances, genetic mutations, and dopaminergic dysfunction. Iron is crucial to dopaminergic pathways in regions like the substantia nigra and basal ganglia. Deficiencies contribute to dopaminergic dysfunction, a key factor in RLS. Genetic mutations reported in BTBD9, MEIS1, PTPRD, and MAP2K5/SKOR1 affect iron transport and dopamine synthesis.17-19
Brain iron deficiency also influences glutamatergic neurotransmission, which is being explored as a therapeutic target. Medications targeting glutamate receptors or modulating dopamine release show promise. Additionally, iron deficiency alters adenosine receptor function, increasing hyperdopaminergic and hyper glutamatergic states worsening RLS symptoms.20-26
RLS is linked to periodic leg movements (PLM), disrupted sleep, and potentially increasing cardiovascular risks. It is common in chronic kidney disease and dialysis patients.27-48 RLS often coexists with ADHD, sleep disorders, and IBS, requiring a multidisciplinary approach. Treatment should be initiated when symptoms significantly affect daily functioning.49-56 However, there is limited data on RLS in the Indian population, and no study on SNP analysis from India, making identification and treatment of the condition more challenging.
MATERIAL AND METHODS
Patients coming to the Department of Pulmonary Medicine OPD were screened for RLS as per the clinical proforma (Annexure 1).
Out of 100 patients, 50 were suspected of having RLS and selected. However, cases with co-morbidities such as diabetes mellitus and neurological conditions were excluded as they did not meet the inclusion criteria. After the vetting process, there were 36 RLS cases and 50 healthy adult controls [Table 1].
Parameters | Case (n=36) | Control (n=50) | p-value |
---|---|---|---|
Gender (Male) | 27 | 22 | 0.000034 |
Gender (Female) | 9(25%) | 28(56%) | 0.000167 |
Age | 56.80 ± 11.19 | 39.38 ± 12.54 | <0.00001 |
RLS severity (mean) | 14.44 ± 6.46 | --- | -- |
For this study, we included 36 cases and 50 controls. There is a statistically significant difference between age and gender for case and control. Mean IRLSSG rating was 14.44 ± 6.46. p-value <0.05 is statistically significant. RLS: Restless legs syndrome, IRLSSG: International Restless Legs Syndrome Study Group.
Inclusion and exclusion criteria
Inclusion criteria for cases were (i) a patient diagnosed according to IRLSSG criteria & (ii) a patient aged between 18-70 years. The exclusion criteria for cases were (i) pregnant women, (ii) patients with an active malignancy, (iii) patients with a history of coronary artery disease, (iv) patients with a history of chronic kidney disease, diabetes, (v) patients who require hemodialysis, and (vi) patients who do not give consent.
Inclusion criteria for the controls were (i) age-matching with the RLS patients, (ii) patients without any chronic diseases, (iii) informed consent. The exclusion criteria for controls were (i) Pregnant women, (ii) patients with pre-existing conditions such as AIDS, Parkinson’s disease, and coronary artery disease as per clinician’s guidelines, and (iii) patients who do not give consent.
Materials
Participants were included based on the IRLSSG criteria, with only adults over the age of 18 being registered. Individuals with chronic illnesses, diabetes, or kidney disease were excluded from the study. For the control group, adults over 18 years old who did not have any chronic diseases, were not pregnant and did not have diabetes mellitus were included.
A hundred patients were screened, of which 50 suspected RLS cases were selected. However, further examination found that some of them had comorbidities (Diabetes mellitus and some neurological problems) and weren’t fulfilling our inclusion criteria and were excluded. After the vetting process there were 36 RLS cases and 50 healthy adult controls.
Biochemical parameters
Serum for LFT (liver function test), RFT (renal function test), and iron profile (Ferritin, TIBC, UIBC, Total Iron, iron saturation) were measured on auto analyzers, (AU680 Beckman Coulter), whereas ferritin was measured using chemiluminescence auto analyzer at the Department of Biochemistry, All India Institute of Medical Sciences (AIIMS) Bhopal, Madhya Pradesh, India [Table 2].
Parameter | Case (n=36) | Control (n=50) | p-value | Reference range |
---|---|---|---|---|
Iron(µg/dL) | 58.23 ± 18.235 | 111.99 ± 31.262 | <0.00001 | 60-180 |
TIBC (µg/dL) | 363.21 ± 60.147 | 325.61 ± 58.047 | 0.004563 | 250-400 |
Iron saturation (%) | 15.23 ± 5.29 | 33.89 ± 11.61 | <0.00001 | 15-50 |
Ferritin (ng/mL) | 67.91 ± 42.01 | 199.23 ± 85.73 | <0.00001 | 10-291 |
ALT (U/L) | 26.48 ± 14.15 | 26.33 ± 7.94 | 0.948521 | <35 |
AST (U/L) | 26.58 ± 9.39 | 19.58 ± 5.32 | 0.000034 | <35 |
ALP (U/L) | 89.59 ± 48.68 | 82.50 ± 20.02 | 0.355851 | 30-120 |
GGT (U/L) | 24.93 ± 9.1 | 22.11 ± 9.26 | 0.164516 | <38 |
In-direct bilirubin (mg/dL) | 0.60 ± 0.31 | 0.62 ± 0.14 | 0.689789 | 0.20-1.0 |
Direct bilirubin (mg/dL) | 0.17 ± 0.10 | 0.19±0.05 | 0.187581 | <0.2 |
Total protein (g/dL) | 7.14 ± 0.56 | 7.15 ± 0.55 | 0.914356 | 6.6-8.3 |
Albumin (g/dL) | 4.26 ± 0.28 | 4.4 ± 0.22 | 0.019478 | 3.5-5.2 |
Globulin (g/dL) | 2.87 ± 0.42 | 2.75 ± 0.55 | 0.288785 | 1.9-3.7 |
A:G ratio | 1.34 ± 0.35 | 1.66 ± 0.40 | 0.000234 | 1-1.7 |
Urea (mg/dL) | 21.19 ± 6.36 | 24.32 ± 8.06 | 0.056575 | 20.0-40.0 |
Creatinine (mg/dL) | 0.94 ± 0.15 | 1.05 ± 0.09 | 0.000029 | 0.5-0.9 |
Sodium (mmol/L) | 135.94 ± 4.03 | 133.78 ± 3.54 | 0.010041 | 136-145 |
Chloride (mmol/L) | 101.36 ± 4.85 | 101.74 ± 3.05 | 0.658636 | 98-107 |
Potassium (mmol/L) | 4.56 ± 0.50 | 4.42 ± 0.47 | 0.176666 | 3.5-5.1 |
The differences between the serum iron profile for cases and controls was determined, which includes serum iron (p-value <0.05), TIBC (p-value <0.05), iron Saturation (p-value <0.05) and ferritin (p-value <0.05), and all were statistically significant. TIBC: total iron-binding capacity, ALT: alanine aminotransferase, AST: aspartate aminotransferase, ALP: Alkaline phosphatase, GGT: gamma-glutamyl transferase, A:G: Adenine: Guanine
DNA extraction protocol from blood
DNA extraction was done using the manual extraction method. Genetic polymorphism testing of genes was detected. The detection of SNPs was carried out by amplification refractory mutation system polymerase chain reaction (ARMS PCR). It is a specialized polymerase chain reaction (PCR) technique used for the detection of specific genetic mutations or SNPs.
Statistical analysis
The data was collected using MS Excel, and the statistical analysis was performed using SPSS software version 21 (IBM, Chicago, IL, US) and GraphPad Prism. The student’s t-test was performed to evaluate the differences between age, gender, and biochemical parameters. Analysis of variance (ANOVA) test was employed to evaluate the differences between the polymorphisms and biochemical parameters. Linear regression was performed to evaluate the possible impact and correlation between the IRLSSG score and serum ferritin. Allele frequency was calculated using the chi-square test. All biochemical values were mentioned as mean ± standard deviation (SD). P-values less than 0.05 reflect statistically significant results. Risk analysis between biochemical parameters and genetic factors will be assessed using odds ratio (OR) calculated with 95% confidence interval (CI).
RESULTS
In our study, we initially screened 100 patients and selected 50 potential cases. However, after further evaluation, some patients were found to have co-morbidities like diabetes mellitus (n=3), hemolyzed sample (n=1), and neurological issues (n=10) that did not meet our inclusion criteria and were subsequently excluded. After this vetting process, the final case group consisted of 36 patients, with 50 healthy adult controls. For data analysis, a t-test was used to evaluate demographic and clinical parameters. Linear regression was employed to examine the relationship between RLS scores and serum ferritin levels, ANOVA was conducted to assess the correlation between alleles and clinical parameters, and the OR was calculated to determine the risk of RLS associated with different SNP alleles under study. Additional parameters assessed included LFTs and RFTs. The LFTs conducted were alkaline phosphatase (ALP), aspartate aminotransferase (AST), alanine aminotransferase (ALT), gamma-glutamyl transferase (GGT), bilirubin (direct/indirect), albumin, globulin, and the albumin-to-globulin (A:G) ratio.
Statistically significant differences were observed between the case and control groups in AST, albumin, and A:G ratio, each with a p-value of less than 0.05. For the RFTs, serum levels of sodium, chloride, potassium, urea, and creatinine were measured. Significant differences between the case and control groups were found in sodium and creatinine levels, both with p-values less than 0.05 [see Tables 1-3].
MEIS1 | ||||
---|---|---|---|---|
Parameter | TT | TG | GG | p-value |
Iron | 56.25 ± 16.90 | 59.1 ± 19.05 | - | 0.672738 |
TIBC | 343.74 ± 48.77 | 371.77 ± 63.51 | - | 0.202219 |
Iron saturation | 16.43 ± 5.03 | 14.70 ± 5.42 | - | 0.374118 |
Ferritin | 54.54 ± 19.51 | 73.79 ± 47.93 | - | 0.209894 |
RLS rating | 14.45 ± 5.95 | 14.44 ± 6.80 | - | 0.995149 |
SNPs: Single nucleotide polymorphisms, TIBC: Total iron binding capacity, RLS: Restless legs syndrome
Linear Regression model analysis
Figure 1 shows a linear regression model comparing RLS scoring with serum ferritin levels. We can see that as serum ferritin levels rise, the RLS scoring decreases establishing an inverse relation between the two.
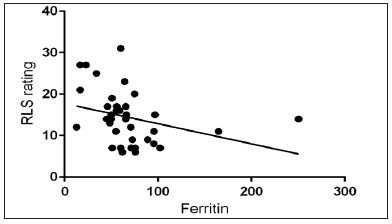
- Interrelationship between RLS rating and Ferritin. RLS: Restless legs syndrome.
Genotype distribution of SNPs among the healthy and case subjects’ analysis:
Supplementary Figure 1a-e presents the genotype distribution of five SNPs among healthy (blue) and affected (orange) individuals. Tables 3-5 outline the RLS-related polymorphisms and their correlations with various biochemical parameters. In this analysis, we examined the differences in clinical parameters across different alleles of polymorphisms and assessed their statistical significance.
For the MEIS1 gene [Table 3], we evaluated both heterozygous and homozygous alleles, but no statistically significant associations were observed. The BTBD9 gene was not analyzed further due to the predominant presence of the heterozygous TG allele in the study population.
In the case of TOX3 [Table 4], we compared the homozygous TT allele, heterozygous TG allele, wild-type GG allele, and the combined (TT+TG) group with the GG allele. However, no statistically significant differences were found in clinical parameters across these comparisons.
TOX3 | ||||
---|---|---|---|---|
Parameter | TT | TG | GG | p-value |
Iron | 57.11 ± 19.04 | 58.83 ± 11.55 | 57.94 ± 26.78 | 0.977203 |
TIBC | 352.62 ± 62.42 | 366.35 ± 60.90 | 364.80 ± 62.59 | 0.878567 |
Iron saturation | 15.34 ± 6.19 | 14.72 ± 3.11 | 15.99 ± 7.58 | 0.826636 |
Ferritin | 64.85 ± 35.75 | 74.39 ± 53.10 | 59.25 ± 21.30 | 0.640598 |
RLS rating | 14 ± 7.50 | 13.88 ± 5.64 | 15.63 ± 7.50 | 0.774026 |
SNPs: Single nucleotide polymorphisms, TIBC: Total iron binding capacity, RLS: Restless legs syndrome
For the PTPRD gene [Table 5], the low frequency of the TT homozygous allele led us to combine it with the TG heterozygous group for comparison against the wild-type CC allele. Again, no significant differences were detected. Similarly, an analysis of the MAP2K5 gene was not conducted due to the predominant distribution of the GA heterozygous allele in the population.
PTPRD | |||
---|---|---|---|
Parameter | TT+TC | CC | p-value |
Iron | 58.58 ± 19.65 | 56.46 ± 9.07 | 0.799409 |
TIBC | 358.15 ± 60.12 | 388.49 ± 58.64 | 0.265423 |
Iron saturation | 15.22 ± 5.75 | 15.27 ± 2.00 | 0.982538 |
Ferritin | 66.54 ± 41.08 | 74.76 ± 50.02 | 0.668117 |
RLS rating | 14.43 ± 6.36 | 14.5 ± 7.63 | 0.982014 |
SNPs: Single nucleotide polymorphisms, TIBC: Total iron binding capacity, RLS: Restless legs syndrome
DISCUSSION
This study highlights the critical role of iron in RLS. It shows a marked decline of iron levels in RLS patients compared to healthy controls, suggesting that low iron may be a factor in the onset of secondary RLS. Variations in serum iron, total iron-binding capacity, iron saturation, and ferritin levels suggest a strong connection between iron metabolism and RLS.20,22 Gender and age differences were observed between RLS cases and controls.6 Although previous studies suggest a higher prevalence of RLS in women, ours found a higher proportion of males in the RLS cohort. However, due to the limited number of confirmed RLS cases, the study could not perform a thorough gender-based analysis.
It was observed that the genotype distribution analysis for specific SNPs highlighted MEIS1, TOX3, and PTPRD as potential genetic factors associated with RLS. Genotype distribution analysis for specific SNPs revealed that genes like MEIS1, TOX3, and PTPRD might contribute to RLS susceptibility. Both cases and controls showed heterozygous mutations, with the T allele predominating [Supplementary Figure 2]. While no distinct at-risk alleles were identified in this cohort, the presence of one copy of the allele in both cases and controls suggests a potential genetic risk factor for RLS. The low iron levels (iron deficiency) may be the primary trigger for RLS, with the identified genetic variants in cases possibly exacerbating the disease. Screening for altered iron profiles, especially in older individuals, may be useful for predicting the likelihood of developing RLS. Iron supplementation could be used as a preventive measure in those with deficiency.23
The study also reviewed genetic variants that affect iron transport, dopamine regulation, and neuroprotection of dopaminergic neurons. Key genes involved in these processes include BTBD9 (chromosome 6p21.2), MEIS1 (chromosome 2p14), PTPRD (chromosome 9p24.1-p23), and MAP2K5/SKOR1, all of which play significant roles in iron metabolism and dopamine biosynthesis [Table 6].4,17-19
Gene | SNP | ALLELE | p-value | OR | 95% CI |
---|---|---|---|---|---|
MEIS1 | rs2300478 | GG* vs. TG | 0.9702 | 1.0784 | 0.0206 to 56.3943 |
GG vs. TT | 0.7251 | 2.0435 | 0.0381 to 109.6845 | ||
GG vs. TG+TT | 0.8718 | 1.3836 | 0.0268 to 71.3588 | ||
TG vs. TT | 0.1508 | 1.9360 | 0.7862 to 4.7677 | ||
BTBD9 | rs9296249 | CC* vs. TC | 0.8796 | 1.3562 | 0.0263 to 69.9594 |
CC* vs. TT | 0.6705 | 3.0000 | 0.0190 to 473.1018 | ||
CC vs. TC+TT | 0.8718 | 1.3836 | 0.0268 to 71.3588 | ||
TC vs. TT | 0.6299 | 2.2121 | 0.0876 to 55.8717 | ||
TOX3 | rs3104767 | TT* vs. TG | 0.9121 | 0.9398 | 0.3122 to 2.8296 |
TT vs. GG | 0.2583 | 0.4773 | 0.1324 to 1.7210 | ||
TT vs. TG+GG | 0.6160 | 0.7644 | 0.2675 to 2.1843 | ||
TG vs. GG | 0.2099 | 0.5078 | 0.1761 to 1.4647 | ||
PTPRD | rs1975197 | AA* vs. GA | 0.3318 | 3.3571 | 0.2910 to 38.7359 |
AA vs. GG | 0.783 | 0.6667 | 0.0372 to 11.9362 | ||
AA vs. GA+GG | 0.3951 | 2.884 | 0.2512 to 33.0706 | ||
GA vs. GG | 0.0574 | 0.1986 | 0.0375 to 1.0521 | ||
MAP2K5 | rs1026732 | AA* vs. GA | 1.0000 | 1 | 0.0136 to 73.2695 |
AA vs. GG | 0.8656 | 1.4058 | 0.0272 to 72.5906 | ||
AA vs. GA+GG | 0.8718 | 1.3836 | 0.0268 to 71.3588 | ||
GA vs. GG | 0.7365 | 1.4118 | 0.1894 to 10.5221 |
RLS is particularly prevalent in individuals with end-stage renal disease (ESRD), affecting up to 68% of patients. It significantly influences their sleep, quality of life, and cardiovascular health.57,58 Ethnic and age-related differences are seen in ESRD populations with RLS, especially in those with chronic kidney disease (CKD), particularly among individuals on hemodialysis or peritoneal dialysis.59 Clinical findings in this study revealed significant differences in iron-related parameters, LFTs, and RFTs between RLS cases and controls.
Although this study found a link between genetic and biochemical markers and RLS, it faced limitations due to the small sample size. Genotypic analysis indicated that certain SNPs might be associated with RLS, but the strength of this association remains unclear. Previous studies have shown that genetic predisposition plays a major role in early-onset RLS, with more than 60% of cases having a positive familial history. There is no information regarding SNP-RLS from India, but variants in MEIS1, BTBD9, and MAP2K5/SKOR1 are known to increase the risk of RLS in some populations [Table 7].60-62
Name of the gene (SNP ID) | Comparison | OR | p-value | 95% CI |
---|---|---|---|---|
MEIS1 (rs2300478) | GG v/s TG | 1.0784 | 0.9702 | 0.0206 to 56.3943 |
GG v/s TT | 2.0435 | 0.7251 | 0.0381 to 109.6845 | |
GG v/s TT+TG | 1.3836 | 0.8718 | 0.0268 to 71.3588 | |
TG v/s TT | 1.9360 | 0.1508 | 0.7862 to 4.7677 | |
BTBD9 (rs9296249) | CC v/s TC | 1.3562 | 0.8796 | 0.0263 to 69.9594 |
CC v/s TT | 3.0000 | 0.6705 | 0.0190 to 473.1018 | |
CC v/s TC+TT | 1.3836 | 0.8718 | 0.0268 to 71.3588 | |
TC v/s TT | 2.2121 | 0.6299 | 0.0876 to 55.8717 | |
TOX3 (rs3104767) | TT v/s TG | 0.9398 | 0.9121 | 0.3122 to 2.8296 |
TT v/s GG | 0.4773 | 0.2583 | 0.1324 to 1.7210 | |
TT v/s TG+GG | 0.7644 | 0.6160 | 0.2675 to 2.1843 | |
TG v/s GG | 0.5078 | 0.2099 | 0.1761 to 1.4647 | |
PTPRD (rs1975197) | AA v/s GA | 3.3571 | 0.3318 | 0.2910 to 38.7359 |
AA v/s to GG | 0.6667 | 0.783 | 0.0372 to 11.9362 | |
AA v/s GA+GG | 2.884 | 0.3951 | 0.2512 to 33.0706 | |
GA v/s GG | 0.1986 | 0.0574 | 0.0375 to 1.0521 | |
MAP2K5 (rs1026732) | AA v/s GA | 1 | 1.0000 | 0.0136 to 73.2695 |
AA v/s GG | 1.4058 | 0.8656 | 0.0272 to 72.5906 | |
AA v/s GA+GG | 1.3836 | 0.8718 | 0.0268 to 71.3588 | |
GA v/s GG | 1.4118 | 0.7365 | 0.1894 to 10.5221 |
The presence of different alleles and their overall risk of RLS. RLS: Restless legs syndrome.
The pilot study offers valuable insights into the demographic, clinical, and genetic aspects of RLS in Indian people. Iron deficiency and genetic factors contribute significantly to RLS. Further research with larger subject cohorts is required to better understand the interplay of genetic, environmental, and physiological factors, especially in the Indian population, to enhance early diagnosis and personalized treatment strategies since no genetic study on the Indian population has been reported [Table 8].
Gene | Allele frequency | Global allele frequency* |
---|---|---|
MEIS1 (rs2300478) | T: 70%, G:30% | T:75.8%, G:24.2%a |
BTBD9 (rs9296249) | T: 50%, C:50% | T:75%, G:25%b |
TOX3 (rs3104767) | T:49%, G:51% | T:45%, G:55%c |
PTPRD (rs1975197) | T: 47%, C:53% | G:8%, A:17%d |
MAP2K5 (rs1026732) | G: 98%, A:2% | G:64%, A:36%e |
It shows predominant presence of T allele. a is MEIS1, b is BTBD6, c is TOX3, d is PTPRD and e is MAP2K5 in which allele and Global frequencies are presented
CONCLUSION
RLS, a leading cause of insomnia, is often underdiagnosed. This study explores the role of iron metabolism, LFTs, RFTs, and genetic factors (specifically SNPs), in RLS pathophysiology. Iron deficiency, linked to dopaminergic dysfunction, was found to significantly affect RLS patients compared to healthy controls. Genetic analysis revealed SNPs in MEIS1, TOX3, and PTPRD, suggesting a genetic predisposition to RLS, although no predominant at-risk alleles were found. Screening for iron deficiency and genetic factors, along with iron supplementation, could aid early diagnosis and therapeutic strategies for RLS.
Author contributions
R.C.: Conceptualization, methodology, formal analysis, investigation, resources, writing—original draft preparation, writing—review and editing, visualization, supervision, project administration; A.V.: Methodology, software, validation, data curation, writing—original draft preparation, writing—review and editing; A.K.Y.: Software, validation, data curation, writing—review and editing; J.R.K.: Validation, resources, supervision; S.G.: Formal analysis, writing—review and editing; A.A.J.: Investigation; A.G.: Visualization.
Ethical approval
The research/study approved by the Institutional Review Board at AIIMS Bhopal, number IHEC-PGR/2021/PG/Jan/05, dated 28th August 2024.
Declaration of patient consent
The authors certify that they have obtained all appropriate patient consent.
Financial support and sponsorship
Nil.
Conflicts of interest
There are no conflicts of interest.
Use of artificial intelligence (AI)-assisted technology for manuscript preparation
The authors confirm that there was no use of artificial intelligence (AI)-assisted technology for assisting in the writing or editing of the manuscript and no images were manipulated using AI.
REFERENCES
- Restless legs syndrome/Willis-Ekbom disease diagnostic criteria: Updated international restless legs syndrome study group (IRLSSG) consensus criteria--history, rationale, description, and significance. Sleep Med. 2014;15:860-73.
- [CrossRef] [PubMed] [Google Scholar]
- Restless legs syndrome: Update on pathogenesis. Curr Opin Pulm Med. 2013;19:594-600.
- [CrossRef] [PubMed] [Google Scholar]
- Linking restless legs syndrome with Parkinson’s disease: Clinical, imaging and genetic evidence. Transl Neurodegener. 2012;1:6.
- [CrossRef] [PubMed] [PubMed Central] [Google Scholar]
- Restless legs syndrome: From pathophysiology to clinical diagnosis and management. Front Aging Neurosci. 2017;9:171.
- [CrossRef] [PubMed] [PubMed Central] [Google Scholar]
- Restless legs syndrome and pregnancy: A review. Parkinsonism Relat Disord. 2014;20:716-22.
- [CrossRef] [PubMed] [PubMed Central] [Google Scholar]
- In the clinic Restless legs syndrome. Ann Intern Med. 2015;163:ITC1-11.
- [CrossRef] [PubMed] [Google Scholar]
- Restless legs syndrome-current therapies and management of augmentation. Nat Rev Neurol. 2015;11:434-45.
- [CrossRef] [PubMed] [Google Scholar]
- Sex and the risk of restless legs syndrome in the general population. Arch Intern Med. 2004;164:196-202.
- [CrossRef] [PubMed] [Google Scholar]
- Restless legs syndrome associated with major diseases: A systematic review and new concept. Neurology. 2016;86:1336-43.
- [CrossRef] [PubMed] [PubMed Central] [Google Scholar]
- The prevalence of restless legs syndrome in taiwanese adults. Psychiatry Clin Neurosci. 2010;64:170-8.
- [CrossRef] [PubMed] [Google Scholar]
- Restless legs syndrome in a community sample of korean adults: Prevalence, impact on quality of life, and association with DSM-IV psychiatric disorders. Sleep. 2009;32:1069-76.
- [PubMed] [PubMed Central] [Google Scholar]
- Restless legs syndrome in chinese elderly people of an urban suburb in Shanghai: A community-based survey. Parkinsonism Relat Disord. 2012;18:294-8.
- [CrossRef] [PubMed] [Google Scholar]
- Prevalence of restless legs syndrome in a Japanese elderly population. Parkinsonism Relat Disord. 2009;15:598-601.
- [CrossRef] [PubMed] [Google Scholar]
- Sleep-related disorders among a healthy population in south india. Neurol India. 2012;60:68-74.
- [CrossRef] [PubMed] [Google Scholar]
- When gender matters: Restless legs syndrome. Report of the ‘RLS and woman’ workshop endorsed by the European RLS Study Group. Sleep Med Rev. 2012;16:297-307.
- [CrossRef] [PubMed] [Google Scholar]
- A genetic risk factor for periodic limb movements in sleep. N Engl J Med. 2007;357:639-47.
- [CrossRef] [PubMed] [Google Scholar]
- Genome-wide association study identifies novel restless legs syndrome susceptibility loci on 2p14 and 16q12.1. PLoS Genet. 2011;7:e1002171.
- [CrossRef] [PubMed] [PubMed Central] [Google Scholar]
- Genome-wide association study of restless legs syndrome identifies common variants in three genomic regions. Nat Genet. 2007;39:1000-6.
- [CrossRef] [PubMed] [Google Scholar]
- Brain imaging and networks in restless legs syndrome. Sleep Med. 2017;31:39-48.
- [CrossRef] [PubMed] [PubMed Central] [Google Scholar]
- Update in restless legs syndrome. Curr Opin Neurol. 2010;23:401-6.
- [CrossRef] [PubMed] [PubMed Central] [Google Scholar]
- The role of iron in restless legs syndrome. Mov Disord. 2007;22:S440-8.
- [CrossRef] [PubMed] [Google Scholar]
- Profile of altered brain iron acquisition in restless legs syndrome. Brain. 2011;134:959-68.
- [CrossRef] [PubMed] [PubMed Central] [Google Scholar]
- Guidelines for the first-line treatment of restless legs syndrome/Willis-Ekbom disease, prevention and treatment of dopaminergic augmentation: A combined task force of the IRLSSG, EURLSSG, and the RLS-foundation. Sleep Med. 2016;21:1-11.
- [CrossRef] [PubMed] [Google Scholar]
- Willis-Ekbom disease foundation revised consensus statement on the management of restless legs syndrome. Mayo Clin Proc. 2013;88:977-86.
- [CrossRef] [PubMed] [Google Scholar]
- The treatment of restless legs syndrome and periodic limb movement disorder in adultsean update for 2012: Practice parameters with an evidence-based systematic review and meta-analyses: An American academy of sleep medicine clinical practice guideline. Sleep. 2012;35:1039e62.
- [Google Scholar]
- Thalamic glutamate/glutamine in restless legs syndrome increased and related to disturbed sleep. Neurology. 2013;80:2028-34.
- [CrossRef] [PubMed] [PubMed Central] [Google Scholar]
- Safety and efficacy of rotigotine transdermal patch in patients with restless legs syndrome: A post-hoc analysis of patients taking 1–3 mg/24 h for up to 5 years. Expert Opin Pharmacother. 2013;14:15-25.
- [CrossRef] [PubMed] [Google Scholar]
- Ca2+ channel α2δ ligands: Novel modulators of neurotransmission. Trends Pharmacol Sci. 2007;28:75-82.
- [CrossRef] [PubMed] [Google Scholar]
- Pregabalin versus pramipexole: Effects on sleep disturbance in restless legs syndrome. Sleep. 2014;37:635-43.
- [CrossRef] [PubMed] [PubMed Central] [Google Scholar]
- The role of n-methyl-D-aspartate (NMDA) receptors in pain and morphine tolerance. Minerva Anestesiol. 2005;71:401-3.
- [PubMed] [Google Scholar]
- Oral ketamine: A promising treatment for restless legs syndrome. Anesthesia and Analgesia. 2002;94:1558-9.
- [CrossRef] [PubMed] [Google Scholar]
- A 10-year, longitudinal assessment of dopamine agonists and methadone in the treatment of restless legs syndrome. Sleep Med. 2011;12:440-4.
- [CrossRef] [PubMed] [Google Scholar]
- Treatment of restless legs syndrome with the selective AMPA receptor antagonist perampanel. Sleep Med. 2017;34:105-8.
- [CrossRef] [PubMed] [Google Scholar]
- Targeting hypersensitive corticostriatal terminals in restless legs syndrome. Ann Neurol. 2017;82:951-60.
- [CrossRef] [PubMed] [PubMed Central] [Google Scholar]
- Pivotal role of adenosine neurotransmission in restless legs syndrome. Front Neurosci. 2018;11:722.
- [CrossRef] [PubMed] [PubMed Central] [Google Scholar]
- Adenosine receptors as markers of brain iron deficiency: Implications for restless legs syndrome. Neuropharmacology. 2016;111:160-8.
- [CrossRef] [PubMed] [PubMed Central] [Google Scholar]
- Heterodimeric adenosine receptors: A device to regulate neurotransmitter release. Cell Mol Life Sci. 2006;63:2427-31.
- [CrossRef] [PubMed] [Google Scholar]
- The time structure of leg movement activity during sleep: The theory behind the practice. Sleep Med. 2012;13:433-41.
- [CrossRef] [PubMed] [Google Scholar]
- Motor pattern of periodic limb movements during sleep. Neurology. 2001;57:300-4.
- [CrossRef] [PubMed] [Google Scholar]
- Heart rate and spectral EEG changes accompanying periodic and non-periodic leg movements during sleep. Clin Neurophysiol. 2007;118:438-48.
- [CrossRef] [PubMed] [Google Scholar]
- Periodic leg movements during sleep and wakefulness in narcolepsy. J Sleep Res. 2007;16:333-9.
- [CrossRef] [PubMed] [Google Scholar]
- Different periodicity and time structure of leg movements during sleep in narcolepsy/cataplexy and restless legs syndrome. Sleep. 2006;29:1587-94.
- [CrossRef] [PubMed] [Google Scholar]
- Periodic leg movements in REM sleep behavior disorder and related autonomic and EEG activation. Neurology. 2002;59:1889-94.
- [CrossRef] [PubMed] [Google Scholar]
- Time structure analysis of leg movements during sleep in REM sleep behavior disorder. Sleep. 2007;30:1779-85.
- [CrossRef] [PubMed] [Google Scholar]
- Change in periodic limb movement index during treatment of obstructive sleep apnea with continuous positive airway pressure. Sleep. 2003;26:717-20.
- [CrossRef] [PubMed] [Google Scholar]
- Periodic leg movements and sleepiness in patients evaluated for sleep-disordered breathing. Am J Respir Crit Care Med. 2001;164:1454-8.
- [CrossRef] [PubMed] [Google Scholar]
- Respiratory-related leg movements and their relationship with periodic leg movements during sleep. Sleep. 2014;37:497-504.
- [CrossRef] [PubMed] [PubMed Central] [Google Scholar]
- A randomized, placebo-controlled crossover study with dipyridamole for restless legs syndrome. Mov Disord. 2021;36:2387-92.
- [CrossRef] [PubMed] [PubMed Central] [Google Scholar]
- Impaired short-term plasticity in restless legs syndrome: A pilot rTMS study. Sleep Med. 2018;46:1-4.
- [CrossRef] [PubMed] [Google Scholar]
- Central and peripheral nervous system excitability in restless legs syndrome. Sleep Med. 2017;31:49-60.
- [CrossRef] [PubMed] [Google Scholar]
- Direct comparison of cortical excitability to transcranial magnetic stimulation in obstructive sleep apnea syndrome and restless legs syndrome. Sleep Med. 2015;16:138-42.
- [CrossRef] [PubMed] [Google Scholar]
- Clinical and electrophysiological impact of repetitive low-frequency transcranial magnetic stimulation on the sensory-motor network in patients with restless legs syndrome. Ther Adv Neurol Disord. 2018;11:1756286418759973.
- [CrossRef] [PubMed] [PubMed Central] [Google Scholar]
- The neurophysiology of hyperarousal in restless legs syndrome: Hints for a role of glutamate/GABA. Adv Pharmacol.. 2019;84:101-19.
- [CrossRef] [PubMed] [Google Scholar]
- Non-REM sleep instability in children with restless sleep disorder. Sleep Med. 2020;75:276-81.
- [CrossRef] [PubMed] [Google Scholar]
- Effects of acute dopamine-agonist treatment in restless legs syndrome on heart rate variability during sleep. Sleep Med. 2011;12:47-55.
- [CrossRef] [PubMed] [Google Scholar]
- Restless legs syndrome, insomnia and quality of life in patients on maintenance dialysis. Nephrol Dial Transplant. 2005;20:571-7.
- [CrossRef] [PubMed] [Google Scholar]
- Restless legs syndrome in hemodialysis patients: Association with depression and quality of life. Sleep Breath. 2011;15:311-5.
- [CrossRef] [PubMed] [Google Scholar]
- Clinical and psycho logical aspects of restless legs syndrome in uremic patients on hemodialysis. Am J Kidney Dis. 2003;41:833-9.
- [CrossRef] [PubMed] [Google Scholar]
- Association studies of variants in MEIS 1 BTBD 9 and MAP2K5/SKOR1 with restless legs syndrome in a US population. Sleep Med. 2011;12:800-4.
- [CrossRef] [PubMed] [PubMed Central] [Google Scholar]
- Genetic association studies in restless legs syndrome: Risk variants & ethnic differences. Can J Neurol Sci. 2024;1-16
- [Google Scholar]
- Restless legs syndrome: A continuing search for better diagnostic criteria. Neurol India. 2018;66:1028-30.
- [CrossRef] [PubMed] [Google Scholar]