Translate this page into:
Tumor Anti-angiogenesis: Nano-Arsenal will Kill the Crab
How to cite: Alam F, Akhtar K, Maheshwari V, Hasan M, Tumor Anti-angiogenesis: NanoArsenal will kill the Crab. Future Health. 2023;01; Future Health 2023;1(1):79-86.
Corresponding Author: Dr Feroz Alam, Assistant Professor Address: Department of Pathology, Jawaharlal Nehru Medical College, Aligarh Muslim University (AMU), Aligarh, Uttar Pradesh, India - 202002 ferozalam97@gmail.com
-
Received: ,
Accepted: ,
Abstract
Angiogenesis: the process of developing new blood vessels from the pre-existing ones is a very crucial process in tumor formation, growth, progression and metastasis. The central role of angiogenesis in tumor growth and progression compels targeting tumor vasculature as a therapeutic mean. Nanoparticles have revolutionized the domain of cancer therapeutics, molecularly targeted anti-cancer drugs represents one of the most significant recent advances in clinical oncology. Various types of nano-drug complexes which targets tumor vasculature preferentially, are being increasingly tested for optimal therapeutic efficacy and minimal side-effects. Here, we review some of these anti-angiogenic nano-drug complexes.
Keywords
Anti-angiogenesis
cancer treatment
nanotechnology
active targeting
Introduction
During embryogenesis, endothelial progenitor cells (angioblasts) form a primitive vascular network of small capillaries, a process termed “vasculogenesis.” Further transformations of this vascular network proceed during “angiogenesis” when new vessels are formed from already existing ones. During angiogenesis, the primary vascular network significantly expands due to capillary branching and is transformed into the highly organized vascular net1. Later, the vascular network matures, and capillaries fuse to form bigger vessels, arteries, and veins. Vasculature in healthy adult is very stable and with the exception of few rare events such as cyclical growth of vessels in the ovarian corpus luteum or during pregnancy, angiog-enic activities are rare in adult individuals2. However, in pathological conditions, numerous disorders are caused or characterized by either excessive or insufficient angiogenesis. The best known of these conditions are diseases with abnormal excessive angiogenesis such as cancer, arthritis, chronic inflammation, infectious or autoim-mune diseases, psoriasis, choroidal neovascularization, and others. Conversely, diseases characterized by insufficient angiogenesis are preeclampsia, Alzheimer disease, stroke, amyotrophic lateral sclerosis, diabetic neuropathy, peripheral artery disease, osteoporosis, and ischemic heart disease3. The development of new blood vessels is induced when the net balance of pro- and anti-angiogenic molecules is tipped in favour of angiogenesis, resulting in the 'angiogenic switch'. During tumour growth angiogenesis is induced by a variety of stimuli. Rapid cell proliferation causes hypoxia as oxygen diffusion is limited by tissue growth. Hypoxia is an important stimulus of tumor vessel growth4, which leads to stabilization of hypoxia-inducible factor-1a (HIF-1α) and HIF-1 target genes leading to angiogenesis.
Some key angiogenic activators include VEGF (vascular endothelial growth factor hereafter VEGF), MMPs (matrix metalloproteinases), PlGF (placenta growth factor), FGF (fibroblast growth factor) and HGF (hepatocyte growth factor)5. Endogenous inhibitors of angiogenesis include thrombospondins (THSBs), endostatin, angiostatin and cytokines such as interleukin-126. New blood vessel formation begins with the removal of pericytes from pre-exisiting blood vessels, initiating the degradation of the endothelial cell basement membrane and extracellular matrix (ECM), a process regulated by the matrix metalloproteinases (MMPs). After this degradation, endothelial cells proliferate and migrate until they form unstable microvessels. Mesenchymal cells differentiate into pericytes, which allow the stability of newly formed vessels and establishing blood flow7. This process of angiogenesis is a very crucial step for tumor formation8. However, the tumor vasculature and its Anatomy are distinct compared to normal vessels - (i) tumor vessels lack the hierarchy of arterioles, capillaries and venules; (ii) tumor vasculature is disorganized and tortuous; (iii) vessels in tumors are leakier than their counterpart in normal tissues since TAECs (tumor associated ECs) are not in close contact with pericytes and are loosely connected to basement membrane; (iv) in some circumstances tumor cells may line blood vessels via vasculogenic mimicry; and (v) compared to ECs isolated from non-tumor tissues, several other molecules have been found to be enriched in TAECs indicative of their distinct molecular properties9.
VEGF- Tumor cells stimulate the formation of new blood vessels by means of enhanced production of the major angiogenic growth factor VEGF10, blockade of VEGF or its receptors reduces tumor growth in multiple models11. The intricacies of angiogenesis and tumor growth seem to be coordinated by cross-talk between VEGF, its receptors, and integrins12. VEGF-A is the major angiogenic growth factor and directly stimulates endothelial cells, mainly via its receptor VEGFR-2 (flk-1) or VEGFR-1 (flt-1)13. This interaction could be reverted by the monoclonal anti-VEGF antibody bevacizumab, suggesting a potential therapeutic role14.
Integrins- Integrins are cell surface receptors for extracellular matrix (ECM) proteins that also play a role in cell-cell attachment. Integrins are important regulators for many different cell processes including both vasculogenesis and angiogenesis15. Blood vessel formation is critically dependent on ECM, whereby integrins are the major adhesion receptors to link ECM proteins with the cytoskeleton16. Of the integrins, αvβ3 is one of the most extensively studied, and has an important role in angiogenesis. It binds and activates mMp-2 at the tips of growing blood vessels to help break down the ECM17. The activation of integrins can be triggered by cytokines of malignant tumor, and blocking αvβ3 integrin inhibits tumor angiogenesis as well as blood vessel formation in in-vivo models18. Hence, αvβ3 is a potential target for tumor antiangiogenic therapy.
Anti-angiogenic therapy- As already mentioned, tumor blood vessels are distinct from normal resting blood vessels, and this distinctness makes them a potential candidate for targeted cancer therapy. In order to block tumor growth and development of metastasis, a number of inhibitors targeting the tumor vasculature have been identified in different in-vitro and in-vivo studies19. Anti-angiogenic therapeutic drugs may act by, inhibiting synthesis of angiogenic proteins by cancer cells, neutralizing the angiogenic proteins, blocking the receptors for angiogenic proteins on endothelium, or directly inducing endothelial cell apoptosis. These inhibitors include therapeutic antibodies and small molecules both capable of targeting angiogenic growth factors, such as VEGF and FGF, or angiogenic growth factor receptors, such as VEGFR and PDGFR. The anti-angiogenic efficacy of chemotherapy is better observed when comparatively low doses of a chemotherapeutic agent are administered on a frequent or continuous schedule. This approach, called metronomic chemotherapy refers to the frequent administration of chemotherapeutic agents at doses significantly below the MTD (maximum tolerated dose) with no prolonged drug-free breaks20,21. Studies have also shown that the endothelial cells of newly forming blood-capillaries are highly and selectively sensitive to very low doses of various chemotherapeutic drugs22-25. Despite several advances there still remain several hindrances to treatment of cancer by anti-angiogenic therapy, including low selective toxicity of anti-cancer drugs, high tumor interstitial fluid pressure (IFP) leading to impaired transport of drug, vasculogenic mimicry in solid tumors, toxicities induced by anti-angiogenic therapy, and drug resistance. Novel strategies to overcome these problems are urgently necessary.
Nanotechnology and anti-angiogenesis - The advantage of nanoscale drug delivery systems is their ability to alter the pharmacokinetics and the bio-distribution of the associated therapeutic agents26. Nanotechnology having ability to engineer materials at the scale of nanometer (nm)27, nanoparticles (NPs) with at least one dimension in the range of 1 to 100 nm are widely applied in the field of medicine specially cancer. For their size NPs have unique operating ability in the complex bio-environment to interact at the level of biomolecules28. The unique size, optical, electrical, magnetic, chemical, and ligands carrying properties of nanoparticles can be targeted to the cancer cells with specificity and monitored efficiently with extreme precision in real-time29.
Nanoparticle circulation is selectively facilitated by biological membranes30, surface modification of NPs may prevent opsonization by the reticulo-endothelial system (RES), and facilitates their retention in blood circulation; the enhanced permeability and retention (EPR) effect31. It gives advantage for using nanoparticulate contrast agents in tumor diagnostic MRI, optical imaging, photo-acoustic imaging, as well as NP delivered therapy32,33. For example, polymer-conjugated angiogenesis inhibitor TnP-470 (caplostatin) was found to accumulate selectively in the tumor vessels by the EPR effect and inhibit hyperpermeability of tumor blood vessels34. Certain nanoparticle-conjugated chemotherapeutic agents such as doxorubicin and angiogenic small molecule inhibitors have been shown to preferentially home into tumors by the EPR effect, resulting in selective vascular shutdown and inhibition of tumor growth35,36. Neutrally charged NPs of average diameter of 10-100 nm and molecular weight around 30 kDa, accumulate inside a tumor due to the EPR effect and referred to 'Passive targeting', however passive targeting may be limited in utility for its low tumor specificity, thereby, lower than required concentration at the tumor target37. Desired specificity and concentration hence, may require 'Active targeting' of the nano-vehicle with moieties such as small ligands, antibodies, and biomarkers capable of specific binding to tumor expressed molecular receptors, facilitating efficient tumor uptake, internalization and receptor-mediated endocytosis resulting in elevated concentrations in tumor cells (Figure-1)37. Monoclonal antibodies (mAbs) in their
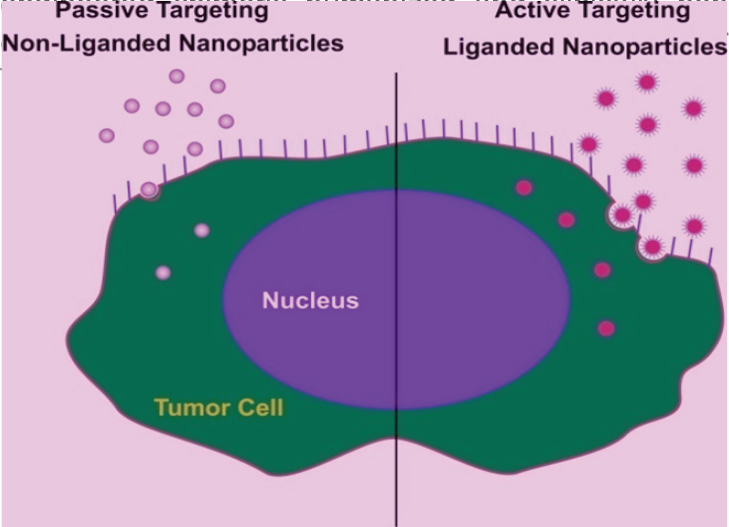
- Showing 'Passive' and 'Active' targeting of nanoparticles
Nanoparticle based anti-angiogenesis - Several nanoparticles have been mediating anti-angiogenic therapy and imaging of the tumor vasculature. These include certain synthetic and few natural nanoparticles, such as polymeric conjugates and polymeric nanoparticles like liposomes and micelles, synthetic organic nanoparticles such as dendrimers, carbon-based nanostructures such as carbon nanotubes and polyhydroxylated fullerenes, inorganic nanoparticles of gold, silver and iron-oxide, quantum dots, viral capsids and ferritin among others.
Polymeric nanoparticles - Polymeric nanoparticles represent one of the most effective nanocarriers for prolonged drug delivery, in the 1980s polyalkylcy-anoacrylate-based nanoparticles releasing doxorubicin led to the development of polymer-based materials for drug delivery38. Langer and Folkman demonstrated the first controlled release of macromolecules using polymers, which allowed the development of antiangiogenic drug delivery systems for cancer therapy and opened new areas for the delivery of macromolecules39. In 1994, nanoparticles composed of poly(lactic acid)/poly (lactic-co-glycolic acid) (PLA/ PLGA) and PEG block copolymer were identified as “long-circulating nanoparticles” due to their stealth properties leading to an increased interest in polymeric nanoparticles and their therapeutic applications40. Several polymeric systems have been shown to inhibit angiogenesis and still others are under investigation.
HPMA copolymers- HPMA copolymers have been extensively studied for their anti-angiogenic potential. The HPMA copolymer was conjugated for the first time to the well known angiogenesis inhibitor TNP-470 (Caplostatin)41. The drug-polymer nanoconjugate selectively accumulated in the tumor microvasculature, leading to decreased tumor growth in human melanoma and lung carcinoma mice models. The drug-polymer nanoconjugate also prevented it from crossing the blood-brain barrier, and preventing neurotoxicity which is a major obstacle in the clinical use of the potent anti-angiogenic drug TNP-470. The action of Caplostatin were attributed to inhibition of various angiogenesis signaling pathways such as VEGF receptor-2 (VEGFR-2), mitogen-activated protein kinase (MaPk) and RhoA42. HPMA copolymers have also been used for bone targeted anti-angiogenic therapy43,44. Specific peptide sequences have been conjugated to HPMA copolymers for active targeting of the avp3 integrin in tumor-associated vasculature. Radionuclide labeled, cyclized RGD peptide-tagged HPMA copolymer based nanoconjugates have been designed that provide the potential for targeted delivery of radionuclides and drugs to solid tumors for diagnostic and therapeutic applications45,46.
Poly(lactic co-glycolic acid) (PLGA) copolymers - Poly(lactic-co-glycolic acid) is a copolymer synthesized from two different monomers glycolic acid and lactic acid47. Sengupta et al. developed a PLGA based nanosystem called as 'nanocell' which was encapsulated within a polyethylene glycol (PEG)-linked lipids envelop48. PEGylation of the nanosystem renders it nontoxic and non-immunogenic, and is an FDA approved method49. To this nanosystem, the chemotherapeutic drug doxorubicin was covalently attached to the inner PLGA core, and the antiangiogenic agent combretastatin was trapped in the outside lipid envelope. The nanocell was destined to disrupt inside the tumor micro-environment and releasing combretastatin, which led to vascular collapse of the tumor. Local hypoxia later, led to the release of doxorubicin, which resulted in significant regression of various tumors including melanoma48. PLGA nanoparticles have also been used for delivering natural products thought to have anti-cancer effects. Curcumin loaded PLGA nanoparticles were reported to successfully suppress tumor necrosis factor (TNF)-regulated expression of VEGF, culminating in reduced tumor metastasis50.
In addition, certain other polymers have been used to construct nanoparticles used to inhibit angiogenesis. Chitosan nanoparticles have shown significant inhibition of tumor growth and induction of tumor necrosis in a mouse hepatocellular carcinoma xenograft model51. Dendrimers (branched polymers) have been used to target VEGF receptors on tumor neovasculature52.
Lipid nanoparticles - Liposomes are self assembling, spherical, closed colloidal structures composed of lipid bilayers that surround a central aqueous space. Liposomes are able to encapsulate lipophilic or hydrophilic drugs within their lipidic layers or in their aqueous core respectively and deliver those to target site for in vivo application. Moreover, liposome delivery system can increase the solubility of hydrophobic drugs and stabilize a variety of therapeutic agents such as peptides, proteins and nucleotides in blood stream53,54. The αvβ3 integrin over-expressed by endothelial cells in the tumor vasculature was targeted for drug delivery using its ligands RGD and the cyclic RGD (cRGD)55. RGD-targeted paclitaxel or doxorubicin-loaded PEGylated liposomes showed superior therapeutic activity over free drug or untargeted liposomes56,57. The antitumor activity of RGD-targeted paclitaxel-loaded liposomes is due to tumor microvessel destruction58. Coupling doxorubicin loaded liposomes with a peptide targeted to bombesin receptors (overexpressed in cancers) improves the therapeutic efficacy of the complex59. A peptide antagonist ATN-161 showed antineoplastic and antimetastatic properties against α5β1 integrin60. ATN-161 conjugated to doxorubicin-loaded PEGylated liposomes increased their therapeutic activity in a melanoma model61. Doxorubicin-loaded PEGylated liposomes functionalized with a peptide were used to target a CD13 isoform overexpressed in the tumor neovasculature62-64. Cationic liposomes can selectively bind and internalize to tumor endothelial cells due to the enrichment of the cell membranes with negatively charged lipids and heparan sulfate proteoglycan65,66. Superior accumulation of oxaliplatin in lung tumors was obtained after intravenous injection of PEG-coated cationic drug-loaded liposomes over neutral liposomes67. Cationic liposomes have also been used for delivery of siRNA against the neoangiogenesis regulator, Argonaute 2 (Ago2) which resulted in Ago silencing in tumors together with apoptosis of tumor blood vessels and decreased tumor growth68,69. In another study, paclitaxel-loaded cationic liposomes (EndoTAG-1) induced endothelial cell apoptosis in vivo, retarded melanoma and pancreatic carcinoma tumor growth, and decreased the number of melanoma lung metastases in vivo70-72. Targeting of tumor vasculature by an aptamer directed against the tumor vasculature marker E-selectin has also been reported73,74. Coupling of a peptide ligand to doxorubicin-loaded liposomes increased doxorubicin accumulation in neuroblastoma leading to destruction of perivascular and endothelial cells and significant increase in survival of neurob-lastoma bearing mice over either endothelial cell targeted or pericyte-targeted liposomes alone75.
Other lipid based nanoparticles used for cancer therapy are the micelles having a core-shell structure. The core of the micelles, which is either the hydrophobic part or the ionic part, can contain small (or bigger) molecules such as therapeutic drugs. The shell provides interactions with the solvent and make the nanoparticles stable in the liquid76. Given their lipophilic nature, most anticancer drugs are inherently water insoluble. By encapsulation of the drug within the hydrophobic core of the micelle, the apparent solubility of the drug can be significantly increased. Hence, micelles allow for the in vivo use of drugs otherwise deemed too hydrophobic or toxic, without having to manipulate the chemical structure of the agent. Additionally, encapsulating the drug within the polymer core affords drug stability by hindering enzymatic degradation and inactivation. Lodamin a novel nano-formulation was developed with the use of poly(ethylene-glycol)-poly(lactic)acid (PEG-PLA) molecules and a very potent anti-angiogenic drug TNP-470 forming a nano-micelle complex which overcame the problems of neurotoxicity, poor bioavailability and short half-life that were associated with the use of this powerful anti cancer drug77. In another study cRGD was conjugated to the outer shell of doxorubicin-loaded polymeric micelles and these modified micelles significantly enhanced their internalization (up to 30-fold) by receptor-mediated endocytosis in tumor endothelial cells overexpressing the αvβ3 integrin78. A micelle based delivery of Hypoxia-inducible factor-1a siRNA (HIF-1α siRNA) was used to treat hypoxic tumors79.
Carbon nanoparticles - Carbon based nanoparticles like nanotubes, nanofibers and fullerenes have gained considerable attention in the field of medicine. Carbon nanotubes (CNTs) are the most studied one, having a basic structure of rolled up seamless cylinders of graphene sheets consisting of a honeycomb of benzene rings in the same plane. Depending on the number of graphene layers from which a single nanotube is composed, CNTs are classified as singlewalled carbon nanotubes (SWNTs) and multi-walled carbon nanotubes (MWNTs)80. CNT-based drug delivery has shown promise for the intracellular delivery of small drug molecules, DNA plasmids, short-interfering RNA (siRNA), and proteins, for cancer therapies in vitro and in vivo81. Carbon nanomaterials are being increasingly studied for their anti-angiogenic potential. Murugesan et al. reported the anti-angiogenic potential of pristine (unmodified) MWNTs, C60 fullerenes and graphite in chick chorioallantoic membrane (CAM) by inhibiting VEGF- and bFGF-induced angiogenesis82. However, these carbon materials did not showed any significant effect on basal angiogenesis in the absence of added growth factors, indicating their differential anti-angiogenic potential only in tumor environment where angiogenic factors are known to be upregulated. In another study, Chaudhuri et al evaluated the effect of doxorubicin conjugated single walled carbon nanotubes (CNT-Dox) and doxorubicin-conjugated spherical polyhydroxylated fullerenes or fullerenols (Ful-Dox) on angiogenesis83. They reported that CNTs exert a proangiogenic effect in vitro and in vivo. In contrast, the fullerenols or doxorubicin-conjugated fullerenols exerted a dramatically opposite antiangiogenic activity in zebrafish and murine tumor angiogenesis models. Other studies have also used carbon nanomaterials for evaluating their anti-angiogenic potential84,85.
Inorganic nanoparticles- Several inorganic nanoparticles, such as those of gold, silver and iron-oxide, possess unique properties, which are increasingly being utilized for biomedical applications, especially cancer diagnosis and treatment. Studies have also demonstrated the anti-angiogenic potential of these inorganic nanoparticles. Bartczak et al. demonstrated deliberate activation or inhibition of in vitro angiogenesis using functional peptide coated gold nanoparticles. The functional particles were shown to influence the extent and morphology of vascular structures, without causing toxicity. Mechanistic studies showed that the nanoparticles have the ability to alter the balance between naturally secreted pro- and anti-angiogenic factors, under various biological conditions86. Studies have also reported similar antiangiogenic properties of silver nanoparticles87-89].
Conclusions
Tumor angiogenesis in recent times has been used as a potential target for therapy and the effects of antiangiogenic therapy has improved the therapeutic index of cancer chemotherapy. The development of targeted cancer therapeutics with improved ability to discriminate between tumor cells and normal cells is one of the major goals of current anti-cancer research. Nanotechnology has presented many innovative methodologies being utilized for targeted drug delivery with minimal toxicities. Hopefully, in future a combinatorial approach of nanotechnology and antiangiogenesis will lead to the development of such drugs which will destroy a large tumor in very short duration with only minimal discomfort and side-effects to the patient.
References
- Mechanisms of Angiogenesis. Biochemistry (Mosc). 2008;73:751-762.
- [CrossRef] [PubMed] [Google Scholar]
- Hypoxia-inducible factor-1 target genes as indicators of tumor vessel response to vascular endothelial growth factor inhibition. Cancer Res. 2008;68:1872-1880.
- [CrossRef] [PubMed] [Google Scholar]
- Targeting tumor angiogenesis with TSP-1-based compounds: rational design of antiangiogenic mimetics of endogenous inhibitors. Oncotarget. 2010;1:662-673.
- [CrossRef] [PubMed] [Google Scholar]
- Tumor angiogenesis: insights and innovations. J Oncol. 2010;2010 132641. https://doi.org/10.1155/2010/132641
- [CrossRef] [PubMed] [Google Scholar]
- Tumor angiogenesis: therapeutic implications. N Engl J Med. 1971;285:1182-1186.
- [CrossRef] [PubMed] [Google Scholar]
- Vasculogenensis, angiogenesis and special features of tumor blood vessels. Front Biosci. 2011;16:1413-1427.
- [CrossRef] [PubMed] [Google Scholar]
- Role of angiogenesis in tumor growth and metastasis. Semin Oncol. 2002;29:15-18.
- [CrossRef] [PubMed] [Google Scholar]
- Vascular endothelial growth factor: basic science and clinical progress. Endocr Rev. 2004;25:581-611.
- [CrossRef] [PubMed] [Google Scholar]
- In Important Advances in Oncology. Lippincott Williams & Wilkins, Baltimore; 1996. p. :69-87.
- [Google Scholar]
- Specificity of vascular endothelial cell growth factor receptor ligand binding domains. Biochem Biophys Res Commun. 1994;201:326-330.
- [CrossRef] [PubMed] [Google Scholar]
- Targeting of VEGF-dependent transendothelial migration of cancer cells by bevacizumab. Mol Oncol. 2010;4:150-160.
- [CrossRef] [PubMed] [Google Scholar]
- Integrins in angiogenesis and lymphangiogenesis. Nat Rev Cancer. 2008;8:604-617.
- [CrossRef] [PubMed] [Google Scholar]
- Definition of two angiogenic pathways by distinct alpha v integrins. Science. 1995;270:1500-1502.
- [CrossRef] [PubMed] [Google Scholar]
- In vitro evaluation of functional interaction of integrin alphavbeta3 and matrix metalloprotease-2. Mol Pharm. 2009;6:1856-1867.
- [CrossRef] [PubMed] [Google Scholar]
- An antagonist of integrin alpha v beta 3 prevents maturation of blood vessels during embryonic neovascularization. J Cell Sci. 1995;108:2655-2661.
- [CrossRef] [PubMed] [Google Scholar]
- Angiogenesis as a therapeutic target. Nature. 2005;438:967-974.
- [CrossRef] [PubMed] [Google Scholar]
- Antiangiogenic scheduling of chemotherapy improves efficacy against experimental drug resistant cancer. Cancer Res. 2000;60:1878-1886.
- [Google Scholar]
- Less is more, regularly: metronomic dosing of cytotoxic drugs can target tumor angiogenesis in mice. J Clin Invest. 2000;105:1045-1047.
- [CrossRef] [PubMed] [Google Scholar]
- The microtubule-affecting drug paclitaxel has antiangiogenic activity. Clin Cancer Res. 1996;2:1843-1849.
- [Google Scholar]
- Protracted low-dose effects on human endothelial cell proliferation and survival in vitro reveal a selective antiangiogenic window for various chemotherapeutic drugs. Cancer Res. 2002;62:6938-6943.
- [Google Scholar]
- Antiangiogenesis is produced by nontoxic doses of vinblastine. Blood. 1999;94:4143-4155.
- [CrossRef] [PubMed] [Google Scholar]
- Paclitaxel at ultra low concentrations inhibits angiogenesis without affecting cellular microtubule assembly. Anticancer Drugs. 2003;14:13-19.
- [CrossRef] [PubMed] [Google Scholar]
- Doxorubicin encapsulated in liposomes containing surface-bound polyethylene glycol: pharmacokinetics, tumor localization, and safety in patients with AIDS-related Kaposi's sarcoma. J Clin Pharmacol. 1996;36:55-63.
- [CrossRef] [PubMed] [Google Scholar]
- What is Nanotechnology? Nanotechnology perceptions Journal (Collegium Basilea). 2005;1:3-17.
- [CrossRef] [Google Scholar]
- Nanotechnology for the biologist. J Leukoc Biol. 2005;78:585-594.
- [CrossRef] [PubMed] [Google Scholar]
- Emerging inorganic nanomaterials for pancreatic cancer diagnosis and treatment. Cancer Treat Rev. 2012;38:566-579.
- [CrossRef] [PubMed] [Google Scholar]
- Clearance properties of nano-sized particles and molecules as imaging agents: Considerations and caveats. Nanomedicine (Lond). 2008;3:703-717.
- [CrossRef] [PubMed] [Google Scholar]
- Nanoparticles in photodynamic therapy: An emerging paradigm. Adv Drug Deliv Rev. 2008;60:1627-1637.
- [CrossRef] [PubMed] [Google Scholar]
- Lessons from multidisciplinary translational trials on anti-angiogenic therapy of cancer. Nat Rev Cancer. 2008;8:309-316.
- [CrossRef] [PubMed] [Google Scholar]
- Exploiting the enhanced permeability and retention effect for tumor targeting, Drug Discov Today. 2006;11:812-818.
- [CrossRef] [PubMed]
- Targeting angiogenesis with a conjugate of HPMA copolymer and TNP-470. Nat Med. 2004;10:255-261.
- [CrossRef] [PubMed] [Google Scholar]
- Cell-penetrating albumin conjugates for enhanced doxorubicin delivery. Polym Chem. 2013;4:4584-4587.
- [CrossRef] [Google Scholar]
- Shape effect of carbon nanovectors on angiogenesis. ACS Nano. 2010;4:574-582.
- [CrossRef] [PubMed] [Google Scholar]
- Theranostic applications of nanomaterials in cancer: Drug delivery, image-guided therapy, and multifunctional platforms. Appl Biochem Biotechnol. 2011;165:1628-1651.
- [CrossRef] [PubMed] [Google Scholar]
- Adsorption of antineoplastic drugs to polyalkylcyanoacrylate nanoparticles and their release in calf serum. J Pharm Sci. 1979;68:1521-1524.
- [CrossRef] [PubMed] [Google Scholar]
- Polymers for the sustained release of proteins and other macromolecules. Nature. 1976;263:797-800.
- [CrossRef] [PubMed] [Google Scholar]
- Biodegradable long-circulating polymeric n anospheres. Science. 1994;263:1600-1603.
- [CrossRef] [PubMed] [Google Scholar]
- Targeting angiogenesis with a conjugate of HPMA copolymer and TNP-470. Nat Med. 2004;10:255-261.
- [Google Scholar]
- Inhibition of vessel permeability by TNP-470 and its polymer conjugate, caplostatin. Cancer Cell. 2005;7:251-261.
- [CrossRef] [PubMed] [Google Scholar]
- Targeting angiogenesis-dependent calcified neoplasms using combined polymer therapeu-tics. PLoS ONE. 2009;4:e5233.
- [CrossRef] [PubMed] [Google Scholar]
- Targeting bone metastases with a bispecific anticancer and antiangiogenic polymeralendronate-taxane conjugate. Angew Chem Int Ed Engl. 2009;48:2949-2954.
- [CrossRef] [PubMed] [Google Scholar]
- Targeting tumor angiogenic vasculature using polymer-RGD conjugates. J Control Release. 2005;102:191-201.
- [CrossRef] [PubMed] [Google Scholar]
- Polymeric conjugates of mono-and bi-cyclic alphaVbeta3 binding peptides for tumor targeting. J Control Release. 2006;114:175-183.
- [CrossRef] [PubMed] [Google Scholar]
- Synthesis and characterization of PLGA nanoparticles. J. Biomater. Sci. Polym. Ed. 2006;17:247-289.
- [CrossRef] [PubMed] [Google Scholar]
- Temporal targeting of tumour cells and neovasculature with a nanoscale delivery system. Nature. 2005;436:568-572.
- [CrossRef] [PubMed] [Google Scholar]
- PEGylation, successful approach to drug delivery. Drug Discov Today. 2005;10:1451-1458.
- [CrossRef] [PubMed] [Google Scholar]
- Design of curcumin-loaded PLGA nanoparticles formulation with enhanced cellular uptake, and increased bioactivity in vitro and superior bioavailability in vivo. Biochem Pharmacol. 2010;79:330-338.
- [CrossRef] [PubMed] [Google Scholar]
- Chitosan nanoparticles inhibit the growth of human hepatocellular carcinoma xenografts through an antiangiogenic mechanism. Anticancer Res. 2009;29:5103-5109.
- [Google Scholar]
- Vascular endothelial growth factor selectively targets boronated dendrimers to tumor vasculature. Mol Cancer Ther. 2005;4:1423-1429.
- [CrossRef] [PubMed] [Google Scholar]
- Nanomedicine:novel approaches in human and veterinary therapeutics. Vet Parasitol. 2011;180:47-71.
- [CrossRef] [PubMed] [Google Scholar]
- Therapeutic opportunities for targeted liposomaldrug delivery. Adv Drug Deliv Rev. 1996;21:117-133.
- [CrossRef] [Google Scholar]
- RGD-based strategies to target alpha(v) beta(3) integrin in cancer therapy and diagnosis. Mol. Pharmaceutics. 2012;9:2961-2973.
- [CrossRef] [PubMed] [Google Scholar]
- RGD-based strategies for improving antitumor activity of paclitaxel-loaded liposomes in nude mice xenografted with human ovarian cancer. J Drug Target. 2009;17:10-8. doi: 10.1080/10611860802368966.
- [CrossRef] [PubMed] [Google Scholar]
- Intracellular delivery of doxorubicin with RGD-modified sterically stabilized liposomes for an improved antitumor efficacy: invitroand invivo. J Pharm Sci. 2005;94:1782-1793.
- [CrossRef] [PubMed] [Google Scholar]
- Integrin-targeted paclitaxel nanoliposomes for tumor therapy. Med Oncol. 2011;28:1180-1187.
- [CrossRef] [PubMed] [Google Scholar]
- Peptide-modified liposomes for selective targeting of bombesin receptors overexpressed by cancer cells: a potential theranostic agent. Int J Nanomedicine. 2012;7:2007-2017.
- [CrossRef] [PubMed] [Google Scholar]
- Pharmacology of the novel antiangiogenic peptide ATN-161 (Ac-PHSCN-NH2): observation of a U-shaped dose-response curve in several preclinical models of angiogenesis and tumor growth. Clin Cancer Res. 2008;14:2137-2144.
- [CrossRef] [PubMed] [Google Scholar]
- Peptide PHSCNK as an integrin alpha(5)beta(1) antagonist targets stealth liposomes to integrin overexpressing melanoma. Nanomedicine. 2012;8:1152-1161.
- [CrossRef] [PubMed] [Google Scholar]
- Enhanced antitumor efficacy of clinical-grade vasculature-targeted liposomal doxorubicin. Clin Cancer Res. 2008;14:7320-7329.
- [CrossRef] [PubMed] [Google Scholar]
- Size-controlled, dual-ligand modified liposomes that target the tumor vasculature show promise for use in drug resistant cancer therapy. J Control Release. 2012;162:225-232.
- [CrossRef] [PubMed] [Google Scholar]
- Structure activity relation-ships of linear and cyclic peptides containing the NGR tumor-homing motif. J Biol Chem. 2002;277:47891-47899.
- [CrossRef] [PubMed] [Google Scholar]
- Cationic liposomes target angiogenic endothelial cells in tumors and chronic inflammation in mice. J Clin Invest. 1998;101:1401-1413.
- [CrossRef] [PubMed] [Google Scholar]
- Phosphatidylserine is a marker of tumor vasculature and a potential target for cancer imaging and therapy. Int J Radiat Oncol Biol Phys. 2002;54:1479-1484.
- [CrossRef] [PubMed] [Google Scholar]
- Kiwada. Oxaliplatin encapsulated in PEG-coated cationic liposomes induces significant tumor growth suppression via a dual-targeting approach in a murine solid tumor model. J Control Release. 2009;137:8-14.
- [CrossRef] [PubMed] [Google Scholar]
- Anti-angiogenic therapy via cationic liposome-mediated systemic siRNA delivery. Int J Pharm. 2012;422:280-289.
- [CrossRef] [PubMed] [Google Scholar]
- Disappearance of the angiogenic potential of endothelial cells caused by Argonaute2 knockdown. Biochem Biophys Res Commun. 2008;368:243-248.
- [CrossRef] [PubMed] [Google Scholar]
- Paclitaxel encapsulated in cationic lipid complexes (MBT-0206) impairs functional tumor vascular properties as detected by dynamic contrast enhanced magnetic resonance imaging. Cancer Biol Ther. 2006;5:89-96.
- [CrossRef] [PubMed] [Google Scholar]
- Neovascular targeting therapy: paclitaxel encapsulated in cationic liposomes improves antitumoral efficacy. Clin Cancer Res. 2003;9:2335-2341.
- [Google Scholar]
- Paclitaxel encapsulated in cationic liposomes: a new option for neovascular targeting for the treatment of prostate cancer. Oncol Rep. 2009;22:321-326.
- [CrossRef] [Google Scholar]
- Thioaptamer conjugated liposomes for tumor vasculature targeting. Oncotarget. 2011;2:298-304.
- [CrossRef] [PubMed] [Google Scholar]
- Aminopeptidase A is a functional target in angiogenic blood vessels. Cancer Cell. 2004;5:151-162.
- [CrossRef] [PubMed] [Google Scholar]
- Combined targeting of perivascular and endothelial tumor cells enhances anti-tumor efficacy of liposomal chemotherapy in neuroblastoma. J Control Release. 2010;145:66-73.
- [CrossRef] [PubMed] [Google Scholar]
- Micellar nanocarriers: pharmaceutical perspectives. Pharm Res. 2007;24:1-16.
- [CrossRef] [PubMed] [Google Scholar]
- An orally delivered small-molecule formulation with antiangiogenic and anticancer activity. Nat Biotechnol. 2008;26:799-807.
- [CrossRef] [PubMed] [Google Scholar]
- cRGD-functionalized polymer micelles for targeted doxorubicin delivery. Angew Chem Int Ed Engl. 2004;43:6323-6327.
- [CrossRef] [PubMed] [Google Scholar]
- Therapeutic Delivery of siRNA Silencing HIF-1 Alpha with Micellar Nanoparticles Inhibits Hypoxic Tumor Growth. Mol Pharmaceutics. 2012;9:2863-2874.
- [CrossRef] [PubMed] [Google Scholar]
- Carbon Nanotubes Innovate on Medical Technology. Med Chem. 2012;2:001-006.
- [CrossRef] [Google Scholar]
- Carbon nanotubes in biology and medicine: An overview. Chin Sci Bull. 2012;57:167-180.
- [CrossRef] [Google Scholar]
- Carbon inhibits vascular endothelial growth factor-and fibroblast growth factor promoted angiogenesis. FEBS Lett. 2007;581:1157-1160.
- [CrossRef] [PubMed] [Google Scholar]
- Comparison of anti-angiogenic properties of pristine carbon nanoparticles. Nanoscale Research Letters. 2013;8:195. http://www.nanosca-lereslett.com/content/871/195
- [CrossRef] [PubMed] [Google Scholar]
- Single-Walled Carbon Nanotubes Mediated Neovascularity Targeted Antitumor Drug Delivery System. J Pharm Pharmaceut Sci. 2013;16:40-51.
- [CrossRef] [PubMed] [Google Scholar]
- Manipulation of in Vitro Angiogenesis Using Peptide-Coated Gold Nanoparticles. ACS Nano. 2013;7:5628-5636.
- [CrossRef] [PubMed] [Google Scholar]
- Antiangiogenic properties of silver nanoparticles. Biomaterials. 2009;30:6341-6350.
- [CrossRef] [PubMed] [Google Scholar]
- Bactericidal silver nanoparticles present an antiangiogenic effect in the Chorioallantoic Membrane Model (CAM) in Science.
- [Google Scholar]