Translate this page into:
In vivo murine models for evaluating anti-arthritic agents: An updated review
*Corresponding author: Dr. Santenna Chenchula, Department of Pharmacology, All India Institute of Medical Sciences, Bhopal, Madhya Pradesh, India. santenna.phd2022@aiimsbhopal.edu.in
-
Received: ,
Accepted: ,
How to cite this article: Chenchula S, Najmi A, Atal S, Sadasivam B. In vivo murine models for evaluating anti-arthritic agents: An updated review. Future Health. 2024;2:138-47. doi: 10.25259/FH_51_2024
Abstract
Arthritis is an autoimmune condition marked by pain, swelling, and stiffness in the joints. Despite significant progress in treatment options, many aspects of arthritis pathogenesis remain poorly understood, necessitating continued research efforts. Animal models have been instrumental in elucidating the complex mechanisms underlying arthritis and in the preclinical evaluation of new treatments. This review article aims to underscore the critical importance of animal models by providing a comprehensive overview of their contributions and current applications in arthritis research. Animal models, such as genetically modified mice and induced arthritis models, offer valuable tools to simulate disease processes observed in humans, enabling researchers to study disease progression, immune responses, and the efficacy of potential therapies in a controlled laboratory setting. The objectives of this review are twofold: initially, to evaluate various types of animal models utilized in arthritis research, highlighting their strengths and limitations, and then, to summarize recent advancements and emerging trends in the field. The present review provides researchers and clinicians with a consolidated resource that informs future research directions and facilitates the translation of preclinical findings into clinical practice.
Keywords
Arthritis
Rheumatoid arthritis
Osteoarthritis
Murine models
In vivo
Autoimmune diseases
INTRODUCTION
Arthritis, characterized by acute or chronic joint inflammation, encompasses over 100 rheumatic diseases and affects more than 5% of the population, with prevalence rising.1 Around 38% of arthritis patients experience disabling symptoms and 42% report severe pain. The four primary types of arthritis are osteoarthritis (OA), rheumatoid arthritis (RA), gout, and ankylosing spondylitis.1,2 Various animal models, including those that develop spontaneously or are induced, are used to study inflammatory joint diseases.3,4 Evaluating antiarthritic agents with these models, which have diverse pathogeneses, allows for rapid assessment of their predictive value, aiding in the development of new treatments.4
RA is a chronic inflammatory autoimmune disease primarily affecting synovial joints.5,6 Effective treatments for arthritis are a major focus in pharmacological research.5 Despite the availability of various disease-modifying antirheumatic drugs and biologics, some patients remain refractory to treatment, highlighting the need for continued research into RA pathogenesis and new therapeutic approaches.5,6 Numerous screening models utilizing both induced and spontaneously developing inflammatory processes are critical for identifying agents that can correct the disease in humans.5 Understanding RA pathogenesis is essential for identifying and evaluating specific drugs.5 Similarly, OA is the most common form of arthritis, particularly affecting the aging population. Chaves HV et al. used a zymosan-induced arthritis model of the temporomandibular joint, resulting in peripheral and central inflammation, pronounced facial mechanical allodynia, and joint pain.6 Murayama et al. developed a rat model of arthritis using rat/hamster antigen-induced arthritis (AIA) and studied cytokine and chemokine gene expression related to angiogenesis.7 They employed quantitative real-time PCR and gelatin zymography to investigate the role of human IL-17A in lymphocyte–macrophage glycosaminoglycan interactions in human OA.7
Animal models
Animal models are indispensable in arthritis research, providing critical insights into disease mechanisms and aiding in the development of new therapeutic strategies. These models can be categorized into induced models, where arthritis is chemically or immunologically induced, and spontaneous models, where arthritis develops due to genetic modifications, as indicated in Table 1.8,9 The aim of this review is to summarize and evaluate the current in vivo studies focusing on the pro-resolving and anti-inflammatory actions of therapeutic agents for arthritis and to highlight the importance of selecting appropriate models to enhance the translational potential of preclinical findings to human clinical applications.
Type of model | Animals involved | Research activity conducted | Outcomes of research | Remarks | References |
---|---|---|---|---|---|
Induced arthritis models | |||||
Collagen-induced arthritis (CIA) | Mice, Rats | Studying the pathogenesis of RA, evaluating therapeutic interventions | Mimics human RA, joint inflammation, cartilage and bone destruction | Most widely used model, high reproducibility | 37,38 |
Adjuvant-induced arthritis (AIA) | Rats | Screening anti-inflammatory drugs, studying chronic arthritis mechanisms | Systemic inflammation, polyarthritis, histological similarities to RA | Useful for drug screening | 39,40 |
Proteoglycan-induced arthritis (PGIA) | Mice | Investigating autoimmune responses, testing biologics | Chronic, erosive polyarthritis, similar immune mechanisms to human RA | Models autoimmune aspect of RA | 41,42 |
Collagen antibody-induced arthritis (CAIA) | Mice | Studying effector phase of arthritis, evaluating rapid onset therapies | Rapid joint inflammation and destruction, bypasses autoimmune priming | Useful for studying effector mechanisms | 43,44 |
Monosodium urate (MSU) crystal-induced arthritis | Mice, Rats | Investigating acute inflammatory response, testing anti-gout therapies | Acute inflammation, neutrophil infiltration, cytokine release | Models acute gout, limited chronicity | 45,46 |
Calcium pyrophosphate dihydrate (CPPD) crystal-induced arthritis | Mice, Rats | Studying pseudogout, evaluating anti-inflammatory drugs | Acute joint inflammation, neutrophil and macrophage activation | Useful for studying pseudogout mechanisms | 47,48 |
Antigen-induced arthritis (AIA) | Rabbits, Rats | Investigating antigen-specific immune responses, testing immunomodulatory drugs | Localized arthritis, reproducible inflammation, cartilage and bone degradation | Models antigen-specific responses | 49,50 |
Zymosan-induced arthritis (ZIA) | Mice | Exploring innate immune responses, evaluating anti-inflammatory compounds | Acute inflammation, macrophage and neutrophil recruitment | Useful for innate immunity studies | 51,52 |
Spontaneous arthritis models | |||||
K/BxN mouse model | Mice | Studying genetic and autoimmune aspects of RA, testing biologics | Spontaneous arthritis, high levels of autoantibodies, chronic joint inflammation | Models autoimmune genetic factors | 53,54 |
TNF transgenic mouse model | Mice | Investigating the role of TNF in arthritis, evaluating TNF inhibitors | Chronic arthritis, joint inflammation and destruction driven by TNF | Useful for studying TNF pathway | 55 |
SKG mouse model | Mice | Exploring genetic predisposition to arthritis, testing immunotherapies | Spontaneous arthritis, strong T-cell involvement, IL-17 dependent | Models genetic susceptibility | 56,57 |
IL-1 receptor antagonist knockout (IL-1Ra KO) mouse model | Mice | Studying the role of IL-1 in arthritis, evaluating IL-1 blockers | Spontaneous arthritis, severe inflammation, IL-1 driven | Models IL-1 mediated pathways | 58,59 |
TNF: Tumor Necrosis Factor, K/BxN: A mouse model of rheumatoid arthritis generated by crossing KRN T-cell receptor (TCR) transgenic mice (K) with non-obese diabetic (NOD) mice (BxN), SKG: A mouse model of autoimmune arthritis caused by a point mutation in the ZAP-70 gene, RA: Rheumatoid Arthritis
Collagen-induced arthritis (CIA)
CIA, or adjuvant arthritis (AA), is the most extensively used and well-characterized model of RA. It is induced by immunizing susceptible rodents, typically mice or rats, by injecting with type II collagen (CII) emulsified in an adjuvant, such as complete Freund’s adjuvant (CFA), which possesses a heat-inactivated mycobacteria at the base of the tail.10 The primary molecular events in CIA pathogenesis involve developing autoimmunity to CII.10 Immunization with CII disrupts immune tolerance, leading to the production of anti-CII antibodies and autoreactive T cells.11 As CII is a crucial structural protein in joint cartilage, it becomes a significant autoantigen in RA. The anti-CII antibodies form immune complexes with CII in the joints, triggering the classical complement pathway, producing inflammatory mediators like C5a, and attracting neutrophils to inflammation sites.12
Activated T cells, B cells, and other immune cells release proinflammatory cytokines such as TNF-α, IL-1β, and IL-6, contributing to synovial inflammation, pannus formation, and cartilage and bone destruction. Chemokines like IL-8 and MCP-1 attract more inflammatory cells to the joint. These cytokines also promote the proliferation and activation of synovial fibroblasts, leading to synovial hyperplasia, while angiogenic factors stimulate new blood vessel formation, exacerbating inflammation.13 Activated synovial fibroblasts and osteoclasts break down cartilage and bone through matrix metalloproteinases and cathepsins, with inflammatory cytokines like RANKL enhancing osteoclast differentiation and activity.14,15
Although CIA replicates many aspects of human RA, the molecular pathways involved are not identical, and the model has limitations in fully capturing the complex etiology and heterogeneity of the human disease. Nevertheless, the CIA remains a valuable tool for studying RA pathogenesis and assessing potential therapies.16
Collagen antibody-induced arthritis (CAIA)
CAIA is a notable model for studying RA, complementing the CIA model [Figure 1]. CAIA is initiated by injecting mice with monoclonal antibodies against specific epitopes on type II collagen (CII), the main protein in joint cartilage.17 This method bypasses the need for active immunization and autoimmunity development required in the CIA.17 The anti-CII antibodies form immune complexes with CII in joints, activating the classical complement pathway.18,19 This activation produces inflammatory mediators such as C5a, which recruits neutrophils and other immune cells. The subsequent release of proinflammatory cytokines—TNF-α, IL-1β, and IL-6—from macrophages and neutrophils drives synovial inflammation, pannus formation, and destruction of cartilage and bone. Chemokines like IL-8 and MCP-1 further attract inflammatory cells to the joints.17,18
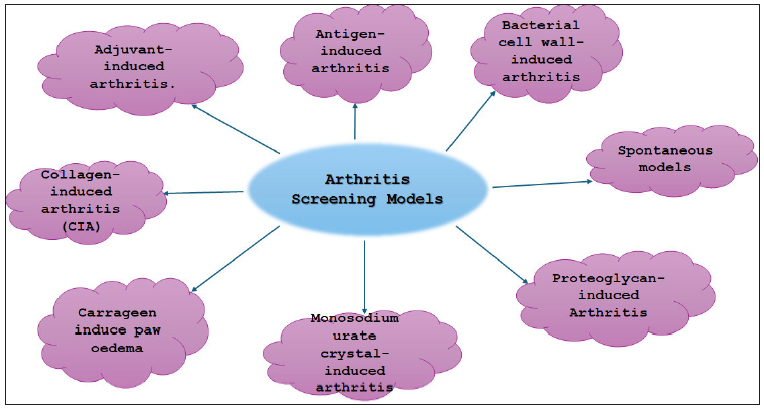
- Various arthritis screening models.
Inflammatory cytokines also stimulate the proliferation of synovial fibroblasts, leading to synovial hyperplasia.20 Angiogenic factors induce new blood vessel formation, worsening inflammation. Activated fibroblasts and osteoclasts degrade cartilage and bone via matrix metalloproteinases and cathepsins, with inflammatory cytokines such as RANKL enhancing osteoclast activity.21-24 The CAIA model offers several benefits, including rapid arthritis induction, avoidance of lengthy immunization, and applicability to mouse strains resistant to CIA, thus allowing study across various genetic backgrounds.25 Unlike the CIA, the CAIA provides a more uniform and predictable disease course but lacks the autoimmune and T-cell-mediated responses seen in the CIA. Despite these limitations, both models are valuable for exploring different disease aspects.26
In CAIA, immune complexes and complement activation trigger the release of proinflammatory cytokines and further attract inflammatory cells.27 Neutrophils and macrophages, activated by these processes, release proteases, reactive oxygen species (ROS), and other mediators that damage joint tissues.28 This model’s ability to bypass autoimmunity development—unlike in CIA—makes it a critical tool for studying arthritis onset and progression.29
Crystal-induced arthritis models
Crystal-induced arthritis is an inflammatory condition of the joints. The primary types of this condition are gout and pseudogout, which result from the accumulation of monosodium urate (MSU) or calcium pyrophosphate (CPP) crystals in the joints.30 Injecting MSU or calcium pyrophosphate dihydrate (CPPD) crystals into mouse or rat joints is a standard method for studying the acute inflammation of gout and pseudogout.30 These crystals are recognized by pattern recognition receptors, including the NLRP3 inflammasome, in immune cells like macrophages.30,31 Activation of the NLRP3 inflammasome leads to the cleavage and release of proinflammatory cytokines IL-1β and IL-18.30 IL-1β and other mediators like leukotriene B4 quickly recruit and activate neutrophils, which release proteases, ROS, and neutrophil extracellular traps, exacerbating the inflammatory response. MSU and CPPD crystals can also directly activate alternative complement pathways, producing inflammatory anaphylatoxins like C5a, further amplifying inflammation.31 Additionally, these crystals stimulate the production of pro-inflammatory eicosanoids, including prostaglandins and leukotrienes, through the activation of phospholipase A2 and cyclooxygenase enzymes.32 The resultant acute inflammation leads to the release of proteolytic enzymes, cytokines, and other mediators, causing synovial inflammation, cartilage degradation, and bone erosion.32
Crystal-induced arthritis models are valuable for studying the initial acute phase of inflammatory arthritis and assessing therapies targeting the NLRP3 inflammasome, eicosanoid pathways, and other mediators of the crystal-induced response. However, they fall short in replicating the chronic and progressive nature of human gout and pseudogout. Despite these limitations, these models offer important insights into the molecular mechanisms of crystal-induced joint inflammation and are crucial for developing effective treatments.33
Proteoglycan-induced arthritis (PGIA)
PGIA is a prominent animal model for studying RA.34 By injecting proteoglycans like aggrecan or decorin into mouse or rat joints, this model simulates the chronic inflammation and joint destruction seen in human RA.34 It is extensively used to investigate RA pathogenesis and evaluate potential therapies.35 In the PGIA model, proteoglycan injection triggers an inflammatory response characterized by T cell and macrophage activation, along with the production of proinflammatory cytokines such as TNF-α and IL-1β. This process leads to cartilage and bone destruction and the formation of pannus tissue, a hallmark of RA.36,37
The PGIA model is used to study various RA aspects, including immune cell roles, cytokine production, and therapeutic efficacy.37,38 It is employed to test the effectiveness of anti-inflammatory drugs like corticosteroids and NSAIDs, as well as biologics such as TNF-α and IL-1β inhibitors.38 Recent advances in PGIA research include new methods for inducing arthritis, such as using recombinant human proteoglycans and targeting specific joints like the knee or ankle.38 The model is also used to assess the impact of environmental factors, including smoking and obesity, on RA development and progression. Despite its limitations in fully representing the genetic and environmental complexity of human RA, the PGIA model remains a valuable tool in preclinical research for understanding disease mechanisms and exploring potential treatments.39
GENETIC MODELS
Transgenic and knockout mouse models of rheumatoid arthritis
Genetically modified mouse models are essential for understanding RA and evaluating new treatments. These models involve altering specific genes to mimic key aspects of human RA. For instance, the TNF-transgenic arthritis model, in which mice overexpress human TNF-α, develops chronic and erosive polyarthritis akin to human RA. This model has been crucial for demonstrating TNF-α’s role in joint inflammation and degradation, leading to the development of anti-TNF therapies. Similarly, the K/BxN serum-transfer arthritis model, which transfers autoantibodies from K/BxN mice to healthy mice, induces rapid and symmetric polyarthritis.40 This model is instrumental for studying autoantibody-mediated inflammation and assessing anti-inflammatory treatments [Table 2].
Type of model | Type of animal used | Specific gene evaluated | Activity performed | Outcomes | Remarks |
---|---|---|---|---|---|
Collagen-induced arthritis (CIA) | Mouse (C57BL/6, DBA/1) | IL-1β, TNF-α, IL-6 | Collagen Type II immunization | Inflammatory arthritis with joint swelling and damage | Widely used, resembles human RA |
K/BxN serum transfer model | Mouse (C57BL/6, BALB/c) | TNF-α, IL-1R | Transfer of arthritic serum from K/BxN mice | Rapid onset of arthritis, joint inflammation | Useful for studying innate immune mechanisms |
TNF-α transgenic mouse | Mouse (C57BL/6, TNF-α TG) | TNF-α | Overexpression of TNF-α gene | Chronic inflammatory arthritis | Mimics chronic inflammation seen in human RA |
IL-1 receptor antagonist knockout (IL-1Ra KO) | Mouse (BALB/c) | IL-1R | Knockout of IL-1Ra gene | Spontaneous arthritis development | Highlights the role of IL-1 in arthritis |
Human TNF-α transgenic (hTNF-α Tg) | Mouse (C57BL/6, hTNF-α Tg) | TNF-α | Expression of human TNF-α gene | Progressive arthritis, joint erosion | Models human RA more closely than murine TNF-α models |
IL-6 transgenic mouse | Mouse (C57BL/6, IL-6 TG) | IL-6 | Overexpression of IL-6 gene | Systemic inflammation, joint swelling | Useful for studying IL-6 pathway in arthritis |
Cartilage oligomeric matrix protein (COMP) KO | Mouse (129Sv) | COMP | Knockout of COMP gene | Development of osteoarthritis | Relevant for studying cartilage-related gene effects |
NF-κB p50 knockout mouse | Mouse (C57 BL/6, p50 KO) | NF-κB p50 | Knockout of NF-κB p50 subunit | Reduced arthritis severity | Highlights the role of NF-κB in inflammation and arthritis |
HLA-B27 transgenic rat | Rat (Lewis, HLA-B27 Tg) | HLA-B27 | Expression of HLA-B27 gene | Development of spondyloarthritis-like symptoms | Models human spondyloarthropathies like ankylosing spondylitis |
Proteoglycan-induced arthritis (PGIA) | Mouse (BALB/c, DBA/1) | IL-12, IL-18 | Immunization with proteoglycan | Severe arthritis with synovial inflammation | Useful for studying immune response to joint antigens |
TNF: Tumor Necrosis Factor, K/BxN: A mouse model of rheumatoid arthritis generated by crossing KRN T-cell receptor (TCR) transgenic mice (K) with non-obese diabetic (NOD) mice (BxN), SKG: A mouse model of autoimmune arthritis caused by a point mutation in the ZAP-70 gene, RA: Rheumatoid Arthritis, IL: Interleukin, KOBALB/c: A strain of inbred laboratory mice used in immunology and cancer research (KOBALB refers to a specific version), DBA: Another inbred mouse strain used for immunological research, including arthritis models
The SKG spontaneous arthritis model features mice with a mutation in the Zap70 gene, a vital T-cell signaling molecule, resulting in spontaneous autoimmune arthritis.41 This model closely resembles the genetic and immunological characteristics of human RA and is used to investigate early disease stages. The IL-1 receptor antagonist knockout model involves deleting the IL-1 receptor antagonist gene, leading to spontaneous, destructive arthritis in mice. This model highlights the IL-1 pathway’s importance in RA pathogenesis and is employed to test IL-1-targeted therapies.42
Recent advancements include humanized mouse models expressing human HLA alleles and immune cells to better replicate RA’s genetic and immunological complexity. The use of CRISPR/Cas9 gene editing allows for precise genetic modifications, improving the accuracy of RA models.43 Combining these models with technologies such as single-cell sequencing and in-vivo imaging offers deeper insights into RA’s cellular and molecular mechanisms.44
K/BxN spontaneous arthritis model
The K/BxN spontaneous arthritis model is a well-established mouse model that mimics key features of human RA. Mice expressing the KRN T-cell receptor (TCR) transgene and the MHC class II molecule Ag7 (K/BxN mice) develop severe erosive polyarthritis spontaneously [Figure 2]. The disease onset in this model involves the recognition of a ubiquitous self-antigen.45 The KRN TCR identifies a peptide from the glycolytic enzyme glucose-6-phosphate isomerase (GPI), which is presented on the Ag7 MHC class II molecule. This recognition activates GPI-specific CD4+ T cells, leading to the production of anti-GPI autoantibodies. These autoantibodies form immune complexes that deposit in the joints, activating the complement system and Fc receptors on innate immune cells.46 This activation recruits and activates inflammatory cells, such as neutrophils and macrophages, which drive synovial inflammation and joint destruction.47 Joint damage is mediated by proinflammatory cytokines, chemokines, and proteolytic enzymes secreted by activated immune cells, directly harming cartilage and bone. Additionally, osteoclast activation and increased bone resorption contribute to the erosive nature of the disease. Recent preclinical studies with the K/BxN model have provided valuable insights into RA pathogenesis and therapy evaluation.47
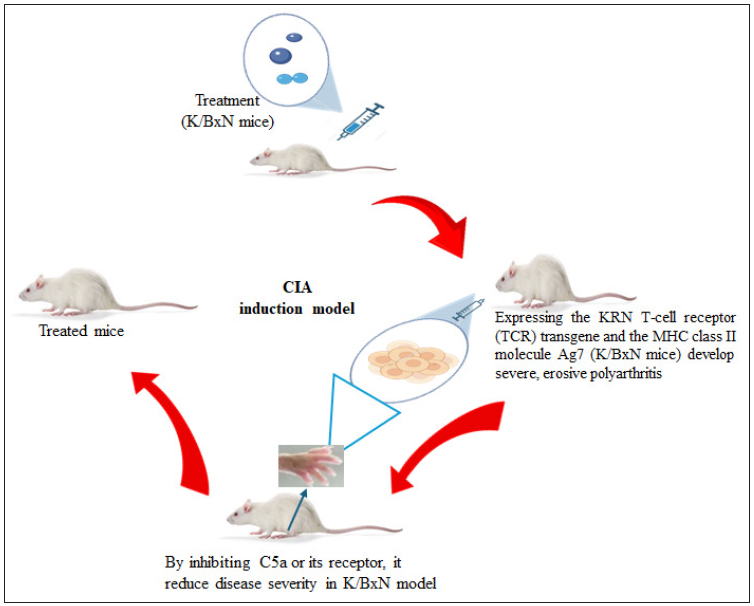
- Schematic representation of K/BxN spontaneous arthritis model. CIA: Collagen-induced arthritis, KRN: T-cell receptor transgenic mice, often used in autoimmune arthritis research, MHC: Major Histocompatibility Complex.
Targeting the complement system, such as by inhibiting C5a or its receptor, has reduced disease severity in the K/BxN model. Strategies to suppress autoreactive T-cell responses, including targeting costimulatory molecules or inducing regulatory T cells, have shown therapeutic promise.48 Further studies have highlighted additional intervention targets, such as the IL-23/IL-17 axis and the NLRP3 inflammasome.48 The K/BxN model has also been used to assess the efficacy of various biologics, small molecules, and combination therapies in preclinical trials. Its ability to replicate autoimmune and inflammatory mechanisms underlying RA makes it a valuable tool for advancing disease understanding and developing new therapies.49
Human TNF-α transgenic (hTNFtg) mice
The hTNFtg mouse model is crucial for studying TNF-α’s role in RA and evaluating TNF-targeted therapies. This model involves genetically modifying mice to express the human TNF-α gene, resulting in systemic overproduction of this proinflammatory cytokine. This genetic modification mimics the elevated TNF-α levels found in RA patients and triggers joint inflammation.50 In the hTNFtg model, mice spontaneously develop chronic, erosive polyarthritis that closely resembles human RA. The arthritis is marked by joint inflammation, synovial hyperplasia, cartilage destruction, and bone erosion, reflecting the progressive nature of the disease in humans.51 Excess TNF-α not only directly drives these inflammatory processes but also stimulates the production of other proinflammatory cytokines, such as IL-1β and IL-6, which further aggravate joint inflammation. Additionally, TNF-α enhances osteoclast differentiation and activation, leading to bone erosion, a key feature of RA. Overall, the hTNFtg model offers valuable insights into TNF-α-mediated pathways in RA and is essential for testing and developing therapies targeting TNF-α and its downstream effects.52
Preclinical studies in hTNFtg mice
The hTNFtg mouse model is crucial for evaluating potential RA therapies. Engineered to overexpress human TNF-α, this model has demonstrated the efficacy of anti-TNF agents like infliximab and etanercept in alleviating arthritis symptoms. These findings directly contributed to the clinical development and approval of anti-TNF therapies for RA.53
The hTNFtg model has also been key in investigating downstream pathways involved in RA.54 For example, inhibiting IL-6 signaling with anti-IL-6 receptor antibodies has shown promise in reducing joint inflammation and damage.54 Similarly, targeting the Janus Kinase (JAK/STAT) Signal Transducer and Activator of Transcription pathway, which mediates cytokine signaling, has been effective in slowing arthritis progression in this model. Studies have explored combination therapies, revealing synergistic effects when combining agents such as anti-TNF and anti-IL-6 therapies.54 This approach underscores the potential benefits of multitarget treatments for enhanced efficacy in RA. Additionally, the hTNFtg model has facilitated research into novel therapeutic targets, including the NLRP3 inflammasome and the IL-23/IL-17 axis, highlighting them as potential future interventions.55,56
Advantages of rodent screening models in arthritis research
Animal models, especially rodent models like CIA, are vital for investigating the mechanisms and pathology of various types of arthritis, including RA and OA. These models replicate key symptoms of human arthritis, such as joint inflammation, tissue destruction, and autoantibody production.57
Beyond studying pathogenesis, animal models are crucial for the preclinical evaluation of new anti-arthritic drugs, biologics, and other treatments before human trials.58 Additionally, these models help predict potential toxicity and assess the safety of novel therapies. Identifying safety concerns early allows for better dosing strategies in human trials.59
Challenges in translating findings from rodent arthritis models to human treatments
Rodent models have been crucial for studying arthritis, particularly RA, but they cannot fully replicate the complexity and variability of human RA.60-63 Differences in disease onset, chronicity, severity, histopathology, and therapy responses highlight their limitations in modeling human disease, which is influenced by diverse genetic and environmental factors.64 The translational success rate from animal studies to human treatments remains low, with many potential therapeutics failing in human trials despite success in animal models.65,66 While rodent models like CIA and adjuvant-induced arthritis are widely used for their ability to mimic key aspects of human RA, they have limitations. They may not fully capture the complexity and heterogeneity of human disease.67 Although rodent arthritis models provide valuable insights, their limitations highlight the need for enhanced human-relevant research methodologies to advance RA treatment strategies and bridge the gap between preclinical findings and successful clinical outcomes.68-73
To address these challenges, there is a need to shift toward research methods that more accurately reflect human immune responses and disease mechanisms.66 Balancing the use of rodent models with an increased focus on human-relevant research approaches is essential for developing more effective therapies tailored to human RA complexities.66,68
Future directions
There is an urgent need for models that more accurately replicate human arthritis, such as humanized mice with human genes linked to the disease and organ-on-chip technology that mimics human joint microenvironments. These innovations can offer a more precise platform for studying disease mechanisms and testing potential therapeutics. Integrating multiple models can also enhance our understanding of arthritis. For example, using both CIA and CAIA models concurrently can help researchers explore both autoimmune initiation and effector phases of RA. Combining mechanical injury models with genetic models may provide insights into post-traumatic OA. Advanced imaging techniques, like MRI and PET scans, enable real-time visualization of disease progression and treatment effects in animal models. Identifying and validating biomarkers that correlate with human disease can improve the prediction of clinical outcomes.
Moreover, developing models that represent various arthritis subtypes and comorbidities, such as cardiovascular disease and metabolic syndrome, can offer a more comprehensive approach to understanding and treating arthritis in patients with multiple health conditions. Adhering to the 3Rs principles—reduction, refinement, and replacement—is crucial for ethical and scientific progress. This involves refining existing models to minimize animal suffering, reducing the number of animals used through better experimental design, and replacing animal models with alternatives like in vitro systems and computational models when feasible.
CONCLUSION
Animal models have been crucial in arthritis research, providing essential insights into disease mechanisms and aiding in the development of potential therapies. Models such as CIA and CAIA have greatly enhanced our understanding of RA, while models for OA and crystal-induced arthritis have shed light on other forms of arthritis. Despite their limitations, these models are vital for preclinical research as they replicate key aspects of human disease. However, inherent limitations, such as differences in genetics, immune system function, and disease pathogenesis between animals and humans, restrict the direct translation of findings. The complexity and heterogeneity of human arthritis cannot be fully captured by any single model, resulting in variability in predicting therapeutic outcomes. Consequently, the success rate of translating discoveries from animal models to effective human treatments remains low.
Ethical approval
Institutional Review Board approval is not required.
Declaration of patient consent
Patient consent not required as there are no patients in this study.
Financial support and sponsorship
Nil.
Conflicts of interest
There are no conflicts of interest.
Use of artificial intelligence (AI)-assisted technology for manuscript preparation
The authors confirm that there was no use of AI-assisted technology for assisting in the writing of the manuscript and no images were manipulated using AI.
REFERENCES
- treasure island (FL): StatPearls Publishing; 2024 [Last accessed 2024 Jul 28]. Available from: https://www.ncbi.nlm.nih.gov/books/NBK518992/
- The use of animal models in rheumatoid arthritis research. J Yeungnam Med Sci. 2023;40:23-29.
- [CrossRef] [PubMed] [Google Scholar]
- Role of preclinical arthritis models for the clinical translation of anti-arthritic therapeutics. Pharm Sci. 2023;29:263-82. Available from: https://doi.org/10.34172/PS.2023.7
- [CrossRef] [Google Scholar]
- Experimental animal models for rheumatoid arthritis. Immunopharmacol Immunotoxicol. 2018;40:193-200.
- [CrossRef] [PubMed] [Google Scholar]
- Mechanism of action of quercetin in rheumatoid arthritis models: Meta-analysis and systematic review of animal studies. Inflammopharmacology. 2023;31:1629-45.
- [CrossRef] [PubMed] [PubMed Central] [Google Scholar]
- Experimental model of zymosan-induced arthritis in the rat temporomandibular joint: Role of nitric oxide and neutrophils. J Biomed Biotechnol.. 2011;2011:707985. doi: 10.1155/2011/707985
- [PubMed] [Google Scholar]
- Chemokines and chemokine receptors as promising targets in rheumatoid arthritis. Front Immunol. 2023;14:1100869.
- [CrossRef] [PubMed] [Google Scholar]
- Animal models of osteoarthritis: Updated models and outcome measures 2016-2023. Regen Eng Transl Med. 2024;10:127-46.
- [CrossRef] [PubMed] [PubMed Central] [Google Scholar]
- Pros and cons of animal models: Special emphasis on anti-arthritic clinical evaluation model. Indian J Pharm Educ Res.. 2023;57:22-7. Available from: https://doi.org/10.5530/001954641253
- [CrossRef] [Google Scholar]
- Collagen-induced arthritis mouse model. Curr Protoc. 2021;1:e313.
- [CrossRef] [PubMed] [Google Scholar]
- Absence of interleukin-17 receptor a signaling prevents autoimmune inflammation of the joint and leads to a Th2-like phenotype in collagen-induced arthritis. Arthritis Rheumatol. 2014;66:340-9.
- [CrossRef] [PubMed] [Google Scholar]
- Complement in the initiation and evolution of rheumatoid arthritis. Front Immunol. 2018;9:1057.
- [CrossRef] [PubMed] [PubMed Central] [Google Scholar]
- HLA-B27 transgenic rat: An animal model mimicking gut and joint involvement in human spondyloarthritides. Ann N Y Acad Sci. 2009;1173:570-4.
- [CrossRef] [PubMed] [Google Scholar]
- Collagen-induced arthritis and imiquimod-induced psoriasis develop independently of interleukin-33. Arthritis Res Ther. 2016;18:143.
- [CrossRef] [PubMed] [PubMed Central] [Google Scholar]
- The mouse model of collagen-induced arthritis. Methods Mol Med. 2004;102:295-312.
- [CrossRef] [PubMed] [Google Scholar]
- The NZB/W F1 mouse model for Sjögren’s syndrome: A historical perspective and lessons learned. Autoimmun Rev. 2020;19:102686.
- [CrossRef] [PubMed] [PubMed Central] [Google Scholar]
- Recent advances and future directions in understanding and treating chlamydia-induced reactive arthritis. Expert Rev Clin Immunol. 2017;13:197-206.
- [PubMed] [Google Scholar]
- Rapid systemic bone resorption during the course of staphylococcus aureus-induced arthritis. J Infect Dis. 2006;194:1597-600.
- [CrossRef] [PubMed] [Google Scholar]
- Efficient promotion of collagen antibody induced arthritis (CAIA) using four monoclonal antibodies specific for the major epitopes recognized in both collagen induced arthritis and rheumatoid arthritis. J Immunol Methods. 2005;304:126-36.
- [CrossRef] [PubMed] [Google Scholar]
- B Cells in rheumatoid arthritis: Pathogenic mechanisms and treatment prospects. Front Immunol7. 2021;12:750753. doi: 10.3389/fimmu.2021.750753
- [Google Scholar]
- Understanding the molecular mechanisms underlying the pathogenesis of arthritis pain using animal models. Int J Mol Sci.. 2020;21:533. doi: 10.3390/ijms21020533
- [CrossRef] [PubMed] [Google Scholar]
- Age/autoimmunity-associated B cells in inflammatory arthritis: An emerging therapeutic target. Front Immunol. 2023;14:1103307. doi: 10.3389/fimmu.2023.1103307
- [CrossRef] [PubMed] [PubMed Central] [Google Scholar]
- Mechanistic insights into the role of B cells in rheumatoid arthritis. Int Immunopharmacol.. 2021;99:108078.
- [CrossRef] [PubMed] [Google Scholar]
- Induction of osteoclastogenesis and bone loss by human autoantibodies against citrullinated vimentin. J Clin Invest. 2012;122:1791-802.
- [CrossRef] [PubMed] [PubMed Central] [Google Scholar]
- Autoantibody epitope spreading in the pre-clinical phase predicts progression to rheumatoid arthritis. PLoS One. 2012;7:e35296.
- [CrossRef] [PubMed] [Google Scholar]
- Identification of a novel chemokine-dependent molecular mechanism underlying rheumatoid arthritis-associated autoantibody-mediated bone loss. Ann Rheum Dis. 2016;75:721-9.
- [CrossRef] [PubMed] [PubMed Central] [Google Scholar]
- Bone loss before the clinical onset of rheumatoid arthritis in subjects with anticitrullinated protein antibodies. Ann Rheum Dis. 2014;73:854-60.
- [CrossRef] [PubMed] [Google Scholar]
- Glycan profiling of anti-citrullinated protein antibodies isolated from human serum and synovial fluid. Arthritis Rheum. 2010;62:1620-9.
- [CrossRef] [PubMed] [Google Scholar]
- Evaluation of anti-nociceptive activity of achyranthes aspera Linn in experimental animal models. Int J Appl Res.. 2015;1:198-201. Available from: https://www.allresearchjournal.com/archives/?year=2015&vol=1&issue=11&part=C&ArticleId=898
- [Google Scholar]
- Bone erosion in rheumatoid arthritis: mechanisms, diagnosis and treatment. Nat Rev Rheumatol. 2012;8(11):656-64. doi: 10.1038/nrrheum.2012.153
- [CrossRef] [PubMed] [Google Scholar]
- Pathomechanisms of bone loss in rheumatoid arthritis. Front Med (Lausanne). 2022;9:962969. doi: 10.3389/fmed.2022.962969
- [CrossRef] [PubMed] [Google Scholar]
- Bone Involvement in Rheumatoid Arthritis and Spondyloartritis: An Updated Review. Biology (Basel). 2023;12(10):1320. doi: 10.3390/biology12101320
- [PubMed] [Google Scholar]
- Bone metabolism in inflammatory arthritis: from mechanisms to therapeutic strategies. Nat Rev Rheumatol. 2020;15:558-66.
- [Google Scholar]
- The pathophysiology of anti-citrullinated protein antibodies in rheumatoid arthritis. Rheumatology. 2021;60:631-42.
- [Google Scholar]
- Mechanisms of disease: shared and individual risk factors for autoimmunity in ACPA-positive and ACPA-negative rheumatoid arthritis. Nat Rev Rheumatol. 2020;16:197-208.
- [Google Scholar]
- Immunisation against heterologous type II collagen induces arthritis in mice. Nature. 1980;283:666-8.
- [CrossRef] [PubMed] [Google Scholar]
- Arthritis in rats induced by adjuvant: a model for human disease. Int Rev Exp Pathol. 1986;28:1-60.
- [PubMed] [Google Scholar]
- Development of arthritis, periarthritis and periostitis in rats given adjuvants. Proc Soc Exp Biol Med. 1956;91:95-101.
- [CrossRef] [PubMed] [Google Scholar]
- Anti-arthritic efficacy of Bombax ceiba ethanolic extract in a murine model for rheumatoid arthritis using in vivo, in vitro and radiological analysis. Bioinformation. 2023;19:833-839.
- [CrossRef] [PubMed] [Google Scholar]
- Antibody-induced arthritis: disease mechanisms and immune regulation. Nat Rev Rheumatol. 2005;1:102-8.
- [Google Scholar]
- How antibodies to a ubiquitous cytoplasmic enzyme may provoke joint-specific autoimmune disease. Nat Immunol. 2002;3:360-5.
- [CrossRef] [PubMed] [Google Scholar]
- Gout-associated uric acid crystals activate the NALP3 inflammasome. Nature. 2006;440:237-41.
- [PubMed] [Google Scholar]
- Crystal ball gazing: New therapeutic targets for hyperuricaemia and gout. Rheumatology (Oxford). 2009;48:222-6.
- [CrossRef] [PubMed] [Google Scholar]
- The value of synovial fluid assays in the diagnosis of joint disease: A literature survey. Ann Rheum Dis. 2002;61:493-8.
- [CrossRef] [PubMed] [Google Scholar]
- Collagen-induced arthritis in mice. Methods Mol Biol. 2010;602:181-92.
- [CrossRef] [PubMed] [Google Scholar]
- Antigen-induced arthritis in mice. I. Induction of arthritis in various strains of mice. Arthritis Rheum. 1977;20:841-50.
- [CrossRef] [PubMed] [Google Scholar]
- Zymosan-induced arthritis: A new model for rheumatoid arthritis research. Arthritis Res Ther. 2020;22:106.
- [CrossRef] [PubMed] [PubMed Central] [Google Scholar]
- Collagen-induced arthritis as a model of rheumatoid arthritis: application to the development and testing of novel therapies. ILAR J. 2007;48:246-58.
- [Google Scholar]
- Organ-specific disease provoked by systemic autoimmunity. Cell. 1996;87:811-22.
- [CrossRef] [PubMed] [Google Scholar]
- Anti-inflammatory and arthritic activity of zaltoprofen compared to piroxicam in murine models. Bioinformation. 2022;18:752-6.
- [CrossRef] [PubMed] [PubMed Central] [Google Scholar]
- Induction of osteoclastogenesis and bone loss by human autoantibodies against citrullinated vimentin. J Clin Invest. 2012;122(5):1791-802. doi: 10.1172/JCI60975
- [CrossRef] [PubMed] [Google Scholar]
- The role of anti-citrullinated protein antibodies (ACPA) in the pathogenesis of rheumatoid arthritis. Cent Eur J Immunol.. 2017;42(4):390-398. doi: 10.5114/ceji.2017.72807
- [PubMed] [Google Scholar]
- Arthritis critically dependent on innate immune system players. Immunity. 2002;16:157-68.
- [CrossRef] [PubMed] [Google Scholar]
- Transgenic mice expressing human tumour necrosis factor: A predictive genetic model of arthritis. EMBO J. 1991;10:4025-31.
- [CrossRef] [PubMed] [Google Scholar]
- Altered thymic T-cell selection due to a mutation of the ZAP-70 gene causes autoimmune arthritis in mice. Nature. 2003;426:454-60.
- [CrossRef] [PubMed] [Google Scholar]
- Autoimmune priming, tissue attack and chronic inflammation - the three stages of rheumatoid arthritis. Eur J Immunol. 2014;44:1593-9.
- [PubMed] [Google Scholar]
- Collagen type II-specific monoclonal antibody-induced arthritis in mice: Description of the disease and the influence of age, sex, and genes. Am J Pathol. 2003;163:1827-37.
- [CrossRef] [PubMed] [PubMed Central] [Google Scholar]
- Collagen type II (CII)-specific antibodies induce arthritis in the absence of T or B cells but the arthritis progression is enhanced by CII-reactive T cells. Arthritis Res Ther. 2004;6:R544-50.
- [CrossRef] [PubMed] [PubMed Central] [Google Scholar]
- Induction of arthritis by single monoclonal IgG anti-collagen type II antibodies and enhancement of arthritis in mice lacking inhibitory FcgammaRIIB. Eur J Immunol. 2003;33:2269-77.
- [CrossRef] [PubMed] [Google Scholar]
- Collagen antibody-induced arthritis. Nat Protoc. 2006;1:2512-6.
- [CrossRef] [PubMed] [Google Scholar]
- Induction of arthritis with monoclonal antibodies to collagen. J Immunol. 1992;148:2103-8.
- [CrossRef] [PubMed] [Google Scholar]
- The importance of IL-1 beta and TNF-alpha, and the noninvolvement of IL-6, in the development of monoclonal antibody-induced arthritis. J Immunol. 2002;169:1459-66.
- [CrossRef] [PubMed] [Google Scholar]
- Utility of animal models for identification of potential therapeutics for rheumatoid arthritis. Ann Rheum Dis. 2008;67:1505-15.
- [CrossRef] [PubMed] [Google Scholar]
- Efficient promotion of collagen antibody induced arthritis (CAIA) using four monoclonal antibodies specific for the major epitopes recognized in both collagen induced arthritis and rheumatoid arthritis. J Immunol Methods. 2005;304:126-36.
- [CrossRef] [PubMed] [Google Scholar]
- Homologous type II collagen induces chronic and progressive arthritis in mice. Arthritis Rheum. 1986;29:106-13.
- [CrossRef] [PubMed] [Google Scholar]
- T lymphocytes in collagen II-induced arthritis in mice. Characterization of arthritogenic collagen II-specific T-cell lines and clones. Scand J Immunol. 1985;22:295-306.
- [PubMed] [Google Scholar]
- Type II collagen autoimmunity in animals and provocations leading to arthritis. Immunol Rev. 1990;118:193-232.
- [CrossRef] [PubMed] [Google Scholar]
- Incidence of arthritis and autoreactivity of anti-collagen antibodies after immunization of DBA/1 mice with heterologous and autologous collagen II. Clin Exp Immunol. 1985;62:639-46.
- [PubMed] [PubMed Central] [Google Scholar]
- Characterization of the antibody response in mice with type II collagen-induced arthritis, using monoclonal anti-type II collagen antibodies. Arthritis Rheum. 1986;29:400-10.
- [CrossRef] [PubMed] [Google Scholar]